Lifecycle Management in Offshore Wind: implications for power network infrastructure
Offshore wind power is rapidly growing as an important source of renewable energy. According to the International Energy Agency, global offshore wind capacity is expected to increase fifteen-fold by 2040, from 23 GW in 2018 to 340 GW in 2040. The rapid growth of offshore wind presents significant challenges for lifecycle management of the asset package.
This article outlines a number of key issues in the lifecycle management of offshore wind assets, and specifically wind turbines, and addresses the implications for power network infrastructure.
For this article, the definition of lifecycle management will be taken as the planning, acquisition, use, maintenance and disposal of the energy infrastructure assets.
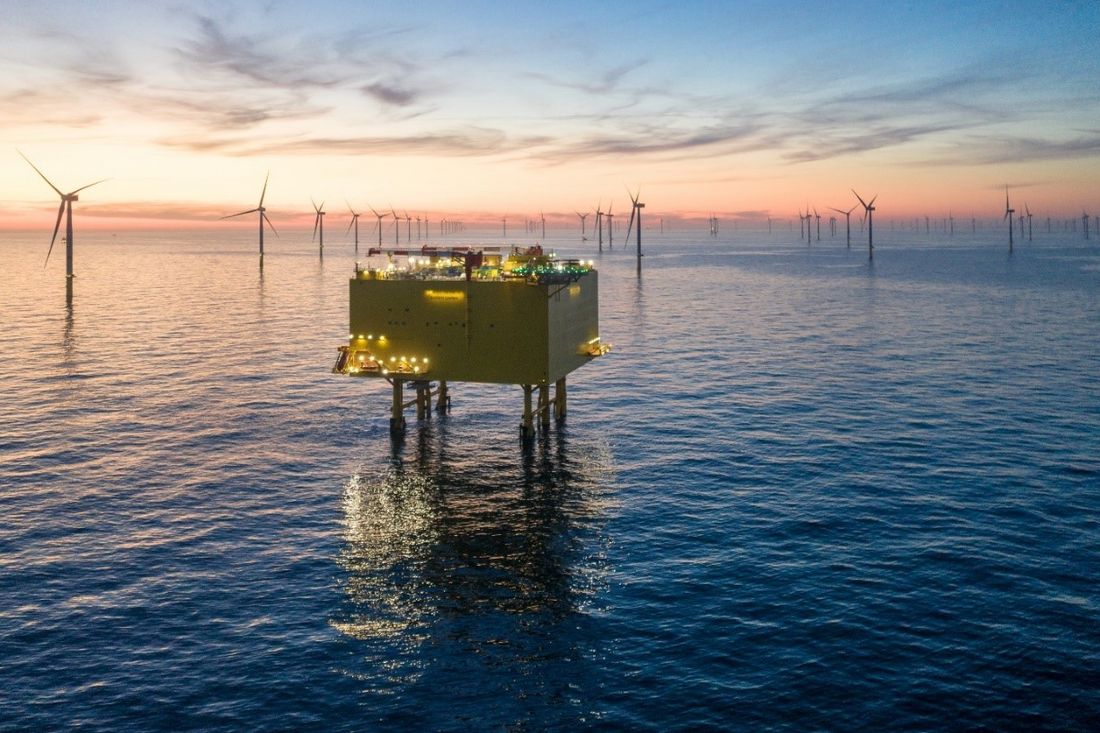
Figure 1 - Offshore wind assets (courtesy: Siemens Energy)
By Adam Middleton, Vice President, Western Europe, for Siemens Energy and Dr. Ray Zhang, Associate Director at ARUP and Professor at the University of Birmingham
Wind Power Market in Europe
Today, turbines of 14 MW and above and being developed and deployed, being some 30 times more powerful than the first machines installed in 1991 in the world’s first offshore wind farm. The current generation of turbines (8 to 10 MW wind turbines) will have blades which measure between 81 and 94 metres in length. The 14MW+ units however will have 115 metre long blades. In terms of the evolution of blade length, it is not the production technology that is the limiting factor, but rather the port infrastructure and offshore installation vessels.
Lifecycle Management in Wind Power
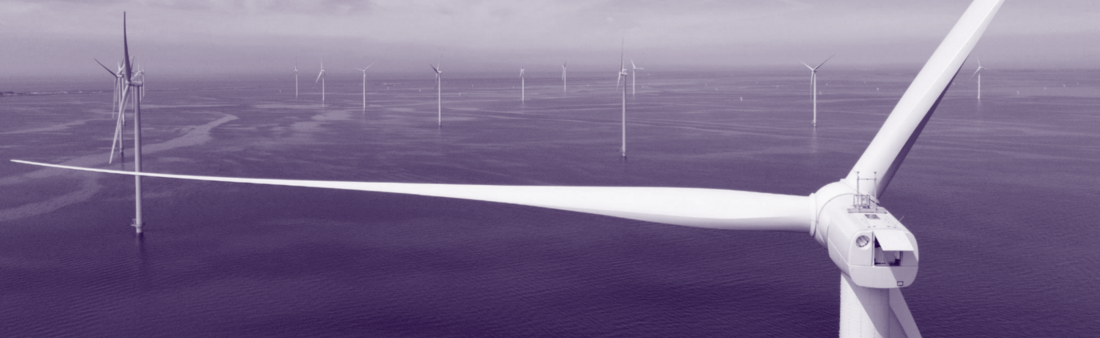
Figure 2 - Offshore Wind Turbine deployment (Courtesy: Siemens Gamesa Renewable Energy)
Although much of the focus of the industry to date has been around development and deployment of larger and more efficient turbines, there is a growing focus upon the environmental implications of the mass deployment of wind, especially offshore.
Effective lifecycle management is critical for the offshore wind industry to ensure safe, reliable, and efficient operations as well as to minimise environmental impacts along the asset lifecycle from design and manufacturing to replacement and disposal. To achieve effective turbine lifecycle management in offshore wind installations, it is necessary to implement a comprehensive maintenance and repair strategy that includes regular inspections and the use of advanced monitoring and diagnostic tools to identify and address potential issues before they become more serious, helping to extend the lifespan of the turbines and ensure that they are operating at maximum efficiency. This also includes the HV network assets that are essential for connecting and transmitting electricity generated by offshore wind farms to the onshore grid.
As part of replacement strategy, life extension is an important aspect of lifecycle management for offshore wind turbines to allow the wind turbine generators (WTGs) to safely and efficiently operate over and beyond their design lifetime. A Life extension assessment, involving a detailed analysis of a turbine’s condition and the remaining useful life of its components is the first step for operators to extend the lifespan of their turbines beyond their original design life. This assessment leads to a comprehensive maintenance plan including year on year detailed tasks (including, for example, additional inspections, monitoring, diagnostics and performance analysis) as well as optional upgrades, refurbishment or retrofits on control systems, drivetrains and blades. Suffice it to say, safety is considered as the key driver for in the calculation of additional years´ of WTG operation.
In addition, it is apparent that after 20 years’ service, some wind farms can continue to be operated, which postpones the recycling phase, especially since a second-hand market for turbines is developing in a number of European countries that allows a second life for many wind turbines.
Lifetime Management and Repurposing of Blades
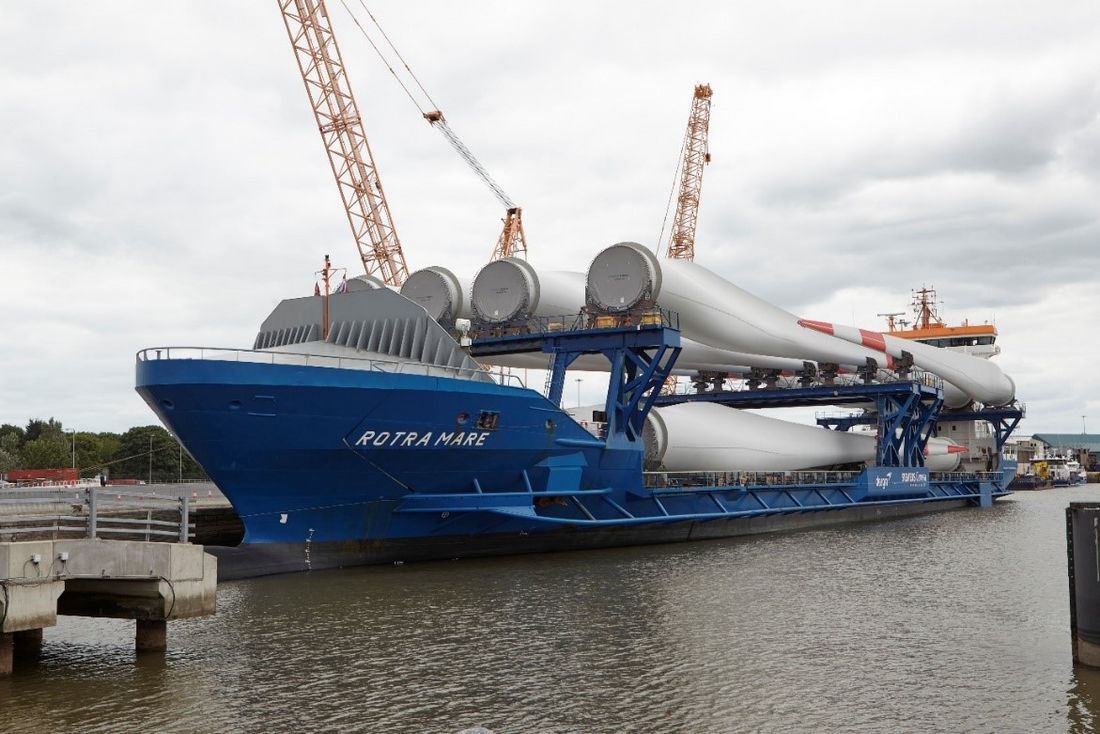
Figure 3 - Recyclable Blade (Courtesy: Siemens Gamesa Renewable Energy)
Today, 85-90% of the total mass of a wind turbine can already be recycled [1]. By 2025, these targets will increase to 95% and 55% respectively, making wind energy one of the industries with the highest recyclability requirements.
Up to now, only the blades have not been recycled but the know how exists on how to recover the materials (for example in the concrete and mortar industry). This covers the use of wind turbine blades shredded and its fiberglass content being used to replace core materials such as sand in the manufacture of cement.
There has been understandable recent outcry in the media regarding disposal of life expired turbine blades and the wind industry is strongly in favour of banning landfilling, which some countries still allow.
In September 2021, Siemens Gamesa Renewable Energy became the first manufacturer to announce the potential of industrially producing recyclable offshore wind turbine blades (“RecyclableBlade”) [2]. From the initial deployment in Germany in 2022, it is anticipated that the blades will reach production in 2025.
The RecyclableBlade employs a new epoxy resin that separates the resin from fiberglass or carbon by immersing the blade in a slightly acidic bath heated to approximately 80 ° C. Subsequently, fibre and resin recovered from the life expired blades can then be reused for new products, for example in the automotive, rail or boating industries.
Blades are not yet 100% recyclable. Fiber and resin account for more than 90% of the blade's weight. The remaining percent is polyurethane used for leading edges, together with paint. A number of technological challenges remain to be overcome to recycle these materials. However, the wind turbine industry remains focused to bring a 100% recyclable blade to market before 2030.
Implications for Power Network Asset Management
Offshore wind development has significant implications on the power network development and asset management. To fully deploy the forecasted offshore wind and achieve the NetZero target by 2050, the traditional radial connection method on its own will not be sufficient to meet the demand of offshore wind connections. Therefore, there is an urgent need for a more efficient and effective solution, and building a coordinated offshore network is one of ways forward. The UK regulator Ofgem and the Electricity Transmission System Operator (ESO) have initiated the Holistic Network Design (HND) program to pursue such coordinated design and development. [3][4]
The offshore wind deployment will also require reinforcement of the onshore network to acquire additional capacity to transmit the electricity. This offshore reinforcement needs to be coordinated with the development of both offshore network and offshore wind in an effective and agile manner. The British government has set out the ambition to connect up to 50GW of offshore generation to the electricity network by 2030. This ambition will require up to 1000 km of additional circuits on its onshore transmission network, and also some significant changes to the current regulatory framework in order to accelerate delivery of large projects. Ofgem has launched the Accelerated Strategic Transmission Investment (ASTI) framework to facilitate the ambition [5].
In addition to onshore and offshore network reinforcement associated with the offshore wind development, many utilities especially European companies are undertaking massive programs to replace aging assets on both Power Transmission and Distribution networks (as the networks were mainly built or re-built post WWII with 40 - 60 years design lifetime). This poses additional challenges to the build and reinforcement of offshore and onshore power networks.
Regarding asset lifecycle management, network assets share many common issues and challenges as those for WTGs. However, there are still some specific issues related the network due to its infrastructure, which need to be particularly considered when dealing with the connection of wind generation. Firstly, as a power network consists of multiple components both primary plant (e.g. such as transformers, cables and circuit breakers) and secondary devices (protection, control and telecommunications), which may be built, installed and maintained by different suppliers/parties. The interoperability between those primary and secondary equipment within the network, as well as with the connected offshore network and WTGs need to be considered at the design and development stage of the lifecycle management. A set of clear and robust Technical/Functional Specifications are the key to ensure the interoperability, it will also play an important role for the maintenance and Post Delivery Support for network operation, covering required parts and spares. The specification will also have big impact on the solutions for replacement and refurbishment in the later stage of network asset lifetime.
Another specific challenge for the network asset management is the system access. With the large scale of onshore and offshore enforcement as well as network replacements, it is rather difficult to get required outages for the installation and commissioning work while a power network is already having heavy congestions/constraints. Smart planning tools, effective collaboration program, innovative technologies such as IEC61850 may offer some solutions to this challenge.
Knowledge and skills management and regulatory framework changes are among other challenges for successfully and timely delivering the required onshore and offshore network for lifecycle management of offshore wind. It is therefore evident that cross-industry coordinated industrial level collaboration will be a key to effective asset lifecycle management among all stakeholders including government, regulator, utilities, manufacturers, consultancy and research organizations.
References
- https://windeurope.org/newsroom/press-releases/wind-industry-calls-for-europe-wide-ban-on-landfilling-turbine-blades/
- https://www.siemensgamesa.com/en-int/explore/journal/recyclable-blade
- “Holistic Network Design Methodology” [Link]
- “Holistic Network Design Follow-up Excise Methodology” [Link]
- https://www.siemensgamesa.com/en-int/explore/journal/recyclable-blade
And with special thanks to Frederic Petit, President - France, Siemens Gamesa Renewable Energy and Pablo de Repáraz, Product Manager – Lifetime Extension, Siemens Gamesa Renewable Energy.