Impact of high penetration of inverter-based generation on system inertia of networks
The worldwide drive to reduce carbon emissions has led to a global effort to accelerate the development and deployment of renewable energy sources (RES). Most of the RES installed in recent times is wind and solar generation (it is noted that hydro generation has long played a major role in certain power systems). These new resources are non-synchronous in nature and are interfaced with the grid through power-electronics and are often termed as inverter-based resources (IBR). The increased uptake of IBR that is displacing conventional coal fired thermal synchronous generation, both at the transmission and distribution level, has widespread impact on the operation, planning and economics of power systems of today and tomorrow. Amongst others, one major challenge with the increased penetration of IBR on the grid is the reduction of synchronous inertia, as this inertia is almost entirely supplied by the conventional synchronous generators that IBRs displace in the energy market.
Convenor
(ZA)
M. RAMPOKANYO
Secretary
(IE)
P. DATTARAY
Secretary
(ZA)
P. KAMERA
J. BEERTEN (BE), I. ARONOVICH (IL), D. CORCORAN (IE), J. BOS (NL), K. O’KEEFFE (IE), N. MODI (AU), I. RAHIMI (CA), R. QUINT (US), P. TIELENS (BE), D. CHAKRAVORTY (GB), M. BARBIERI (IT), J. MATEVOSYAN (US), E.A. JANSSON (SE), N. GUMEDE (ZA), F.M. CAVALCANTI FERREIRA (BR), A. ATALLAH (DE), B. BERRY (GB), C. MISHRA (US), J. ERNST (GB)
Introduction
Synchronous inertia allows the power system to resist changes in system frequency when there is an imbalance between supply and demand and helps maintain a low rate of change of frequency (RoCoF) so conventional frequency containment reserves (FCR) have enough time to arrest the frequency decline/nadir before the under-frequency load shedding (UFLS) limits can be hit. All synchronous rotating machines connected to the power system contribute to this resistance (inertia) with the kinetic energy that is stored in their rotating masses. On the contrary, IBRs do not inherently provide an equivalent response and release of stored energy immediately following an event. As frequency dynamics get faster in power systems with lower inertia, power system operation, particularly frequency control, becomes more challenging. Existing frequency containment and regulation methodologies have been designed based on the implicit assumption that a certain level of inertia would be available and that this would provide sufficient time for these control services to ensure frequency quality requirements are met under both normal and contingency conditions.
In the absence of high penetrations of IBR, the inertia inherent to synchronous generators meant that the dispatch provided by the energy market ensured almost all systems would have inertia far in excess of their minimum requirement without taking any further actions. Therefore, as the inertia is reduced in the future through changes to the economic dispatch, system operators must now ensure that they establish the following:
- The threats posed to their system by reduced inertia
- The level of inertia at which these threats emerge, and the likelihood of inertia being reduced to these levels in the future.
- The potential mitigation measures for these threats either in the form of new services, practices, or wide area system integrity protection schemes.
- The minimum secure level of inertia with the developed mitigations in place, noting that there will be some degree of economic assessment required when determining this minimum level and the mitigation measures to be developed.
While the emergence of renewable generation is a key driver for the work of this Technical Brochure (TB), the TB itself is focused upon the impact of IBRs and not renewable generation or low carbon generation. For example, hydro generation is not a subject of focus for this TB whilst wind (type III and IV), Photovoltaics (PV), Battery Energy Storage System (BESS) and HVDC systems are discussed in more detail. This is not intended to diminish the role that hydro generation has played in the secure operation of many power systems; it is more reflective of the fact that hydro generation is predominantly synchronous in nature and that operators have well established experience with hydro generation where relevant. Furthermore, in a similar fashion, nuclear generation is a key low carbon technology for many systems, but it is not a subject of discussion in the TB as it is synchronous in nature.
This TB focuses on defining and exploring the impact of IBRs upon the inertia of power systems and how utilities have sought to address these impacts through their studies, services, and grid codes. The objective of this Joint working group (JWG) was to advise and formulate philosophies for system operations to deliver the on-going energy transition, identify key challenges associated with large penetrations of IBR on system inertia, define operational measures to manage these challenges in the short-term, provide detailed lists of plausible technological solutions that would help to address some of the challenges either through development of grid code requirements, standards or market products.
The TB is divided into ten chapters. The opening chapters introduce the TB (Chapter 1), define key terms, set out the basis of power system inertia and its relationship with frequency control (Chapter 2) and provide an overview of existing frequency control practices (Chapter 3). Having established this basis, the TB proceeds to outline the key challenges posed by reduce inertia (Chapter 4) and the capabilities of technologies to help mitigate these challenges (Chapter 5) with a particular focus on grid forming converters in (Chapter 6). The TB then reviews recent utility studies into frequency containment requirements (Chapter 7), the adoption of inertia as an ancillary service (Chapter 8) and a summary of other existing policies relevant to frequency containment (Chapter 9). In this summary, we aim to outline some of the key takeaways from each chapter.
Inertia and frequency control
Under existing operational practices inertia is a key component in maintaining the stability of the power system. It enables the power system to resist any changes in the frequency after a sudden imbalance between supply and demand caused by either generator or load disconnection. Synchronous Inertia is defined as the kinetic energy stored in the rotating mass of machines synchronously connected to a power system. However, adequate or high inertia by itself can only reduce the rate at which frequency changes. But, for overall system security adequate frequency control is equally important.
Figure 1 outlines how inertia can be viewed as an instantaneous RoCoF-limiting service that acts to provide time for primary frequency control to arrest the decline in frequency. It is this instantaneous response that distinguishes inertia from fast frequency response (FFR) services. Inertia acts to limit the peak RoCoF the system is exposed to after any imbalance in supply and demand, while FFR acts to limit average RoCoF over a given measurement time (e.g. 500 ms). Understanding the maximum tolerable peak RoCoF and average RoCoF is a key task in determining how fast FFR will need to be for it to be considered supplementary to inertia. It is the view of this TB that only when the control response of an IBR acts on peak RoCoF can it be considered to be ‘virtual’ inertia rather than FFR and it is essential that the true withstand capability of existing network equipment when exposed to both the peak RoCoF and average RoCoF is considered carefully during this assessment.
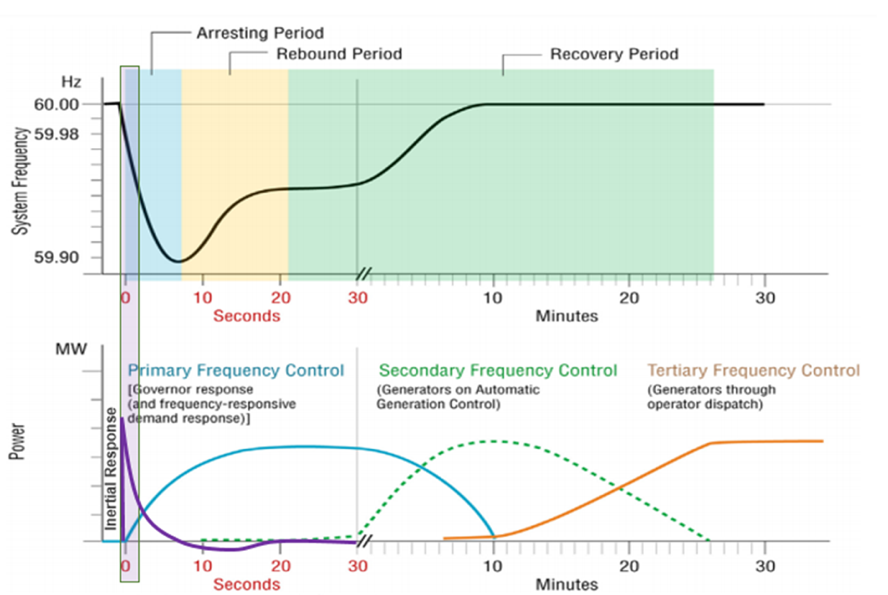
Figure 1 - The sequential actions and impacts of inertia and frequency control
Given the emerging concerns over reduced inertia levels and the move by some utilities to introduce minimum inertia levels or to directly monetize inertia as operational practices, it is inevitable that the estimation of system inertia has received renewed interest. The estimation of system level inertia can be achieved by a number of methods such as status of all online synchronous machines (based on status telemetry), measurement-based techniques using either historical events or continuous estimation using intentional power stimulus, use of high-speed measurement and/or machine learning. Key features of these various techniques are summarized in Figure 2.
At this time, the most widespread method for estimation of power system inertia is using the status of online synchronous machines. It is relatively simple and robust but only estimates the supply side inertia (i.e., it omits the contribution of the demand side generation, non-telemetered generation, and loads), which for many power systems is becoming an important factor in light of reducing inertia levels and increased cost of maintain frequency security.
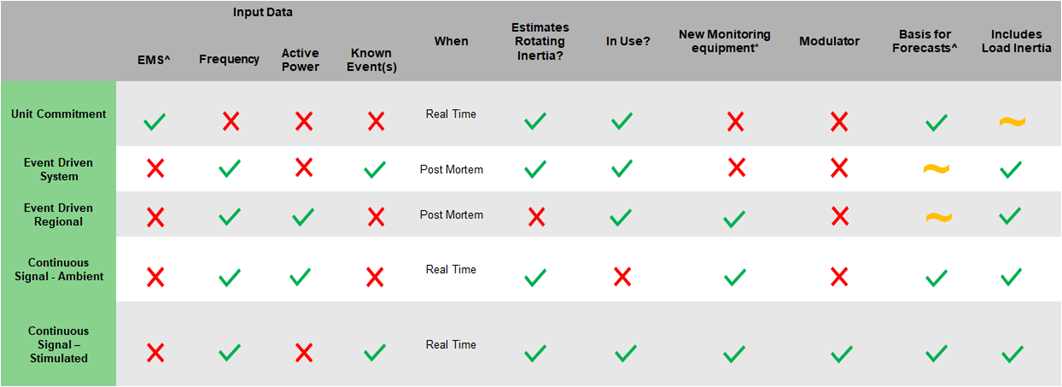
Figure 2 - A summary of inertia estimation methodologies (as this is a constantly evolving area of research, the TB realizes that the functionalities may evolve in time)
^requires status and inertia of units from the EMS
* Requires measurement devices of a specific type or at specific locations
^ The method can be used to forecast inertia for future hours and not just estimate the present level of inertia
Frequency containment reserves, fast frequency response requirements, and minimum inertia levels
The TB outlines the results of a survey of global frequency management practices carried out in 2018/19. This survey highlighted many interesting points about utility practices, one of which was that whilst the challenge of reduced inertia is common to many systems, the specific issues faced by each system are as different as the systems themselves. For example, the maximum permitted RoCoF was found to vary amongst the participating utilities (0.125,0.5,1 and 3 Hz/s) and load shedding can be first initiated for frequency deviations from less than 1% to more than 3% from nominal. This is heavily influenced by system size and the ratio of the system size to the largest possible generator contingency which was found to be highly variable.
Another key outcome was that the assessment of the required FFR/FCR reserves is highly variable across global utilities, the key points of variation include:
- Is the assessment deterministic or probabilistic?
- If the assessment is based on a single event, is it the largest credible contingency at the time under study or a reference incident?
- Does the assessment assume some level of load damping?
- Is FFR/FCR procured from the market or imposed as a requirement?
Finally, whilst many sources continue to discuss the emerging role of IBR in providing FFR/ FCR, the survey results here indicate that over 40% of respondents already depend upon IBRs (wind, BESS or HVDC) for some form of FFR/FCR. This would indicate that some sort of role for IBRs in frequency containment is now approaching the common place.
Challenges associated with increased non-synchronous RES levels
The operation of a power system with large amounts of IBR present unique challenges in terms of maintaining system security and reliability. This is widely discussed in general terms by many sources; so, the focus of the TB is to isolate and discuss specific challenges with a focus on frequency control.
When considering the challenges posed by reduced inertia, it can be helpful to distinguish between those challenges related directly to the loss of inertia and those challenges that emerge because of the more general reduction in synchronous generation e.g., reduction in fault current and reactive power availability. This is desirable as there are different mitigation measures available from different sources for each challenge and it will likely be most efficient when developing solutions to consciously group challenges that can be mitigated in the same way, rather than simply seeking to develop a like for like replacement for the lost synchronous generation.
The key challenges highlighted within the TB are:
- the impact of higher RoCoF on the proper operation of loss of mains (LoM) relays, where it is noted that averaging RoCoF over 500 ms appears to be emerging as a standard practice for the existing RoCoF limits of between 0.5 and 1 Hz/s
- higher RoCoF causing larger frequency deviations before existing containment reserves can fully respond,
- Voltage dip induced frequency dip,
- Underfrequency load shedding (UFLS) and over-frequency generator shedding (OFGS) schemes operate too slowly, at higher RoCoF, frequency may fall too quickly for schemes to operate, or certain relays may block on high RoCoF assuming measurement/instrumentation errors,
- UFLS/OFGS over respond, smaller sized losses may cause their operation, so first stage of these schemes may need to be retuned,
- Increased need for power swing blocking functions on relays,
- With large offshore renewable installations being considered, including the concept of offshore hubs, and large HVDC interties for island systems the size of the largest credible infeed loss in certain systems could often be larger in the future than it is today.
In Figure 3, a good example of the complexity of managing these sometimes-interdependent challenges is presented. This AEMO process for assessing minimum inertia must determine the need for inertia for the largest infeed and outfeed, while considering the ability of FFR to reduce this need for inertia, unless RoCoF constraints begin to bind which themselves vary with inertia. It must also consider the relationship between frequency reserve and inertia (i.e., most sources of inertia provide frequency reserve) and the presence of any special protection schemes.
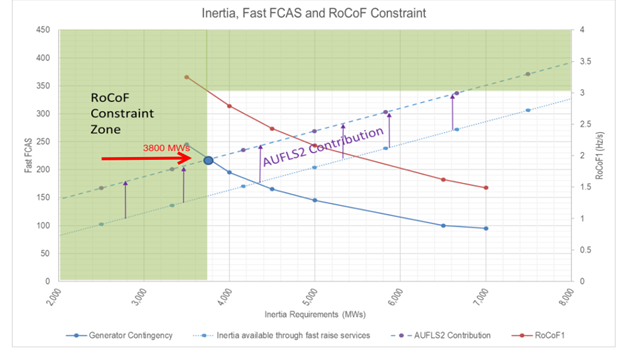
Figure 3 - Relationship between inertia required and FFR to maintain an Acceptable Frequency for the largest Generation Contingency (Fast FCAS refers to Fast Frequency Control Ancillary Service, a type of FFR in Australia)
Capabilities of existing and emerging technologies to provide inertia and frequency response
With a view to mitigating these challenges the capabilities of existing and emerging technologies are then reviewed in the TB. The two key capabilities required to mitigate inertia challenges are:
- Provision of additional inertia
Focused upon the provision of inertia in addition to that which is obtained as a by-product of the results of the energy market and existing reserve markets. - Provision of fast frequency response
New control responses that are defined as fast by virtue of being faster in response than the existing containment reserves.
Additional inertia can be sourced as a service through synchronous condensers or by operating synchronous generation with particularly low minimum stable generation. Furthermore, it may be sourced, in effect, by identifying and quantifying inertia provided by the demand side (e.g., motor loads and private generation) that has always existed but was not quantified when system inertia was higher and accuracy of inertia estimation was not as important.
FFR may be procured from a wide variety of sources and most technologies (e.g., wind, solar, HVDC inter-tie, BESS and synchronous generation) have been reported to be able to provide FFR in some form where conditions allow (e.g., curtailment of solar). In practice utilities appear to mostly depend upon BESS or loads for their FFR at this time. Furthermore, the response requirements that have been specified by utilities for their FFR services appear to vary notably from system to system based on their specific needs in terms of a number of factors, e.g., response time, sustain time and recovery allowance. Finally, for certain large contingencies, it appears relatively common to put in place some form of system integrity protection scheme rather than procuring ancillary services.
The TB discusses characteristics of different existing and future technologies capable of providing inertia, FFR and FCR services and the varied response characteristics of these technologies are highlighted. It should be noted that FCR is a general concept while FFR is a specific utility definition of a set of FCR capabilities that define a service and the only general similarity between FFR services is that they are faster in response than existing FCR services. Thus, FFR in one system may not qualify as FFR in another system. This is inevitable given that FFR addresses a system specific need, and each system will have different needs.
In the TB, it has been found that utilities do not consider synthetic inertia, inertia emulation or other forms of FFR as directly fulfilling part of their inertia requirements. Studies have noted that the availability of FFR can reduce these inertia requirements where the constraining limit is the nadir/zenith. However, the existing FFR services are not assumed to limit the RoCoF measured over 500 ms so they offer no benefit when the inertia is constrained on RoCoF. This may change with the emergence of even faster forms of FFR (almost instantaneous 10-50ms), e.g., from grid forming converters, but it should be noted that any such reduction in RoCoF over 500ms may still need to consider the withstand capacity of equipment. Furthermore, allowing higher RoCoFs may require the measurement of RoCoF over a shorter period of time and it is important to consider the practical challenges of changing the actual RoCoF settings of all impacted devices (e.g., LoM relays) when the RoCoF limit is changed.
In some power systems such as in Ireland and Northern Ireland, GB, ERCOT, South Australia, a minimum inertia constraint is applied. This means that to maintain this constraint additional synchronous generators, beyond what is needed for energy and reserves, may be brought online. Since generators can be brought online for inertia and not for energy, the excess production from synchronous generators operating at their minimum generation level will result in energy curtailment/reduction from cheaper generators (typically wind and solar). Additionally, generators brought online for inertia will need to be compensated at least for their start-up costs and operation at minimum generation level. These are the costs of keeping extra synchronous generators online for inertia.
Operation and possible control strategies for Inverter Based Resources
Grid forming converters are a much-discussed feature of future high-penetration scenarios. In the simplest terms, a grid forming converter is one which establishes its voltage reference (magnitude and frequency) and imposes it on the grid rather than depending upon the grid to provide a voltage and angle reference. This is called self-synchronization and allows a grid forming converter to provide both FFR and a form of virtual inertia (i.e., nearly instantaneous active power injections in response to demand/supply imbalance on the gird).
It is the view here that the term virtual inertia should be preserved for this form of self-synchronized response and not the existing forms of FFR for which the term may have been used in the past. This is because grid forming control of this nature may be able to mitigate RoCoF related issues regardless of the observation window considered, when calculating RoCoF, which is not the case for existing FFR techniques that have inevitable, inherent delays in frequency measurement and response. These FFR responses can only impact the average RoCoF if they respond during the measurement window and will not be able to limit the peak RoCoF. This peak RoCoF may become of more concern in the future as certain studies reviewed in the TB highlighted (a notable increase in the peak RoCoF observed in low inertia systems).
It is important to note that some form of energy storage or buffer is required to deliver the additional current injection to support grid forming functionalities, which incurs additional costs. Furthermore, grid forming control may need to be suspended during fault conditions due to current overload limitations on the converter. Varied control architectures have been proposed to achieve grid forming control and these are discussed in the TB primarily grouped as droop-based control or virtual synchronous machine (VSM).
Quantify frequency containment reserves requirements with increasing IBR penetration
Many utilities are performing detailed studies of their high renewable future to assess their future inertia and containment reserve needs and the TB summarises the study results and methodologies from several studies published by utilities.
The studies summarized, all begin with defining the future system conditions for the study and two general practices can be identified 1) scenario planning and 2) adapting existing operational cases. Scenario planning entails defining one or more future scenarios each of which describes a distinct version of the future and then production cost models are used to generate a year of unit commitments/dispatches to serve as the conditions to study. Figure 4 provides example outputs of a scenario planning process. The TB did not entail an exhaustive review of scenario planning, but it was noted that some utilities invest significant effort into developing long term future scenarios with extensive stakeholder engagement. In contrast, some utilities adapt existing operational cases for their studies, where this adaptation is based on expected changes to the system to ensure the cases cover the future worst cases. In general, adapting operational cases appears to be a less complex task but may be limited to studying near term changes rather than the more radical changes that may occur over the long term.
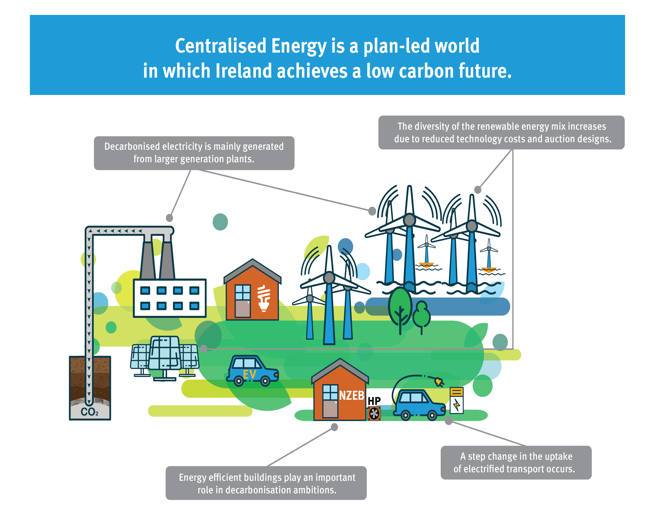
Figure 4 - An example of EirGrid’s future scenarios and South Africa’s renewable plan
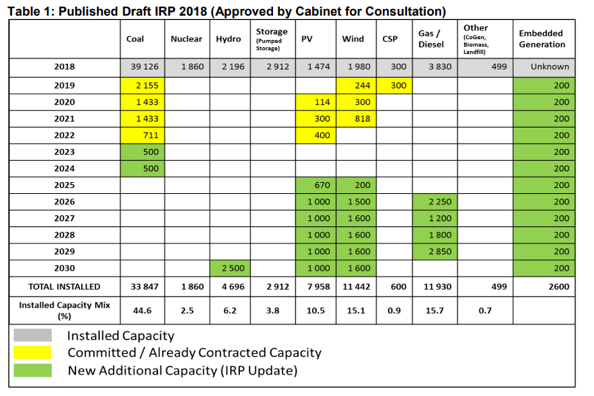
Figure 4 - An example of EirGrid’s future scenarios and South Africa’s renewable plan
The models developed for these studies also differ dramatically in terms of their degree of complexity. Some studies are based on full system models which include detailed models of all synchronous generators, aggregate models of renewable generation and composite load models. In contrast, other studies use ‘single frequency models’ based on the swing equation, model the entire system as a single bus and only include models of the governor/frequency control elements of generators and a simple frequency sensitive load model. Whilst the modelling of demand and its changing nature was outlined in the TB, it is noted that most utilities are still trying to capture the demand side inertia contributions accurately using composite load models as an example with aggregate dynamic motor representations. However, this is not an universal practice amongst the utilities of the world and is subject to constant evolution especially in light of lack of monitoring at the distribution level making load classification and composition estimation particularly challenging.
When performing frequency studies, a key element of any study is the loss of infeed/outfeed for which the system should remain secure. Two practices have been observed here: 1) study a reference incident and 2) study the actual single largest infeed for the hour of operation under study. The reference incident is commonly the total, maximum generation from units/inter-ties connected at a single substation while the single largest infeed is the largest actual dispatch of a generator or HVDC inter-tie. From this review, studies based on the reference incident appear easier to perform although they do result in more conservative results – but it is important to recognize that many utilities that perform reference incident studies are required to by their regulations. Also, most of the studies reviewed consider both loss of infeed events and loss of outfeed events and special schemes are commonly used to deal with specific contingencies.
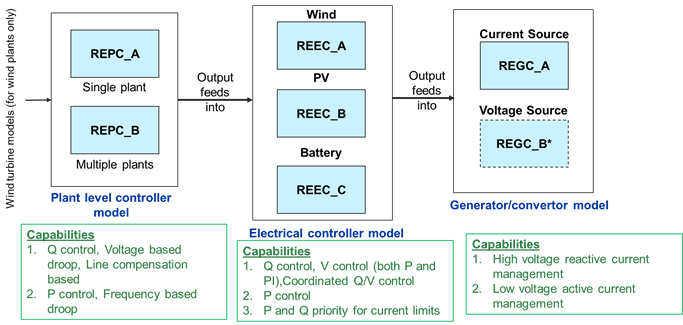
Figure 5 - A 2nd Generation Bulk Power System-connected Renewable energy system (RES) Module that is used in future studies (EPRI) to represent a generic IBR and a simplified model of the entire transmission system used in other studies (EU-SysFlex)
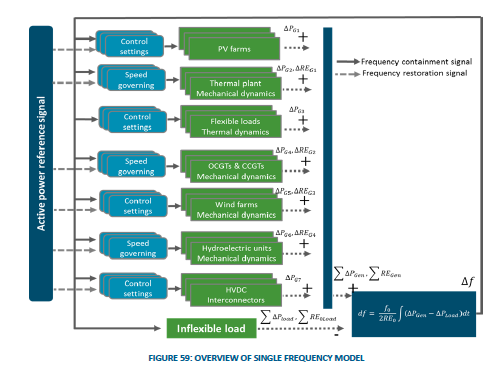
Figure 5 - A 2nd Generation Bulk Power System-connected Renewable energy system (RES) Module that is used in future studies (EPRI) to represent a generic IBR and a simplified model of the entire transmission system used in other studies (EU-SysFlex)
The key outcomes of the studies reviewed in the TB be summarized as:
- The presence and behavior of FFR dominates the results of these studies and can be used to reduce inertia requirements, where the constraint is the nadir/zenith;
- High RoCoF was a concern in several studies, in terms of causing maloperation of LoM relays and exceeding equipment withstand, and impacted utilities only consider inertia when assessing this RoCoF and not the impact of FFR;
- A RoCoF limit/inertia floor is vital to ensuring that the speed of response of existing reserves will remain adequate;
- Some studies have indicated the emergence of regional frequency/RoCoF concerns when large infeed losses occur in low inertia pockets within large interconnected systems;
- Regional inertia floors may be considered when system split is concern;
- Studies become more complex where a wider range of service providers are considered as their response is not equivalent.
Several of these studies have found that the future system is secure with the existing ancillary service definitions. This reflects that these utilities have already developed solutions for their inertia and frequency control challenges, including:
- Inertia floors or RoCoF limits, noting that an inertia floor is equivalent to a RoCoF limit if a reference incident is studied instead of the single largest infeed;
- New FFR services;
- Special schemes to mitigate specific contingencies.
Inertia as an Ancillary Service
The TB explored the use of inertia as an ancillary service. Despite there being a number of power systems around the world that are experiencing a decline in synchronous inertia due to high penetration of inverter-based resources, only a few of these power systems are procuring inertia as a product through various mechanisms. This indicates that for most systems, except smaller islanded systems or systems with specific RoCoF constraints from loss of mains relays, that the energy market continues to implicitly provide sufficient inertia on a system level basis. Although certain existing practices and future study results indicate that in the future greater focus may need to be placed upon ensuring that specific regions of the system have sufficient inertia and not just the system as a whole.
In the procurement phase, it is important to recognize the trade-off between inertia and minimum stable generation level of a synchronous generators providing it, where this trade-off is not implicitly captured by the energy and ancillary services markets, as well as ensure that the resource procured for provision of inertia service complies with any other relevant grid code requirements.
The lack of inertia as an ancillary service in a system with high penetrations of inverter-based resources may mean that these systems must enforce certain inertia requirements at the operational time scale. This may result in sub‑optimal outcomes for both inertia levels and the energy market since in the absence of inertia product, system operators may lack tools for optimizing between start-up costs, minimum generation levels and inertia contribution of the resources.
Existing grid policies around frequency containment reserve requirements in light of higher penetration levels of RES
The TB for this point, draws on a survey of global utilities to provide an overview of the existing grid code policies around the world pertaining to primary frequency response, minimum system inertia, FFR, and other frequency containment measures. The results of this overview are summarised in Figure 6 and indicate that most utilities surveyed have already developed some definition of a minimum inertia level and calculation methodology as well as a FFR service. However, it should be noted that while these efforts are commonplace amongst utilities there are limited parallels between the specific methods and FFR in place within each utility. This reinforces a common theme that whilst these utilities share a common problem from a common source, the specific challenges and solutions depend heavily upon the specific needs and nature of the system in question.
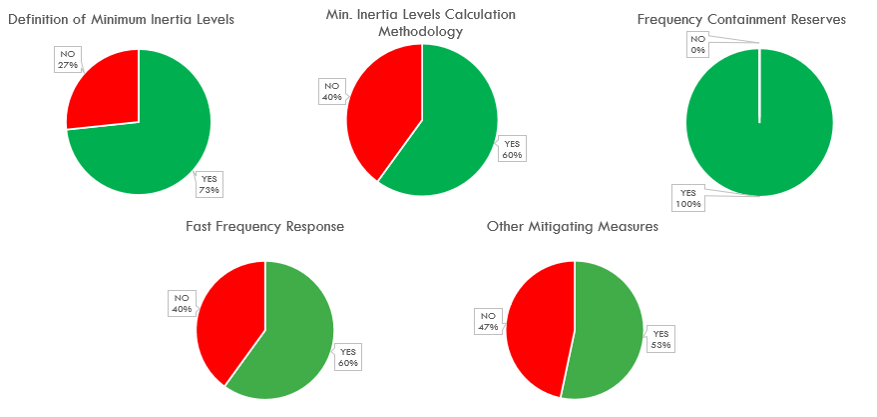
Figure 6 - Summary of key survey results
Conclusions
This TB reviews the challenges posed by reduced power system inertia and the solutions that have been developed or proposed by the industry. The increasing penetration of renewable generation, much of which is inverter based, is an essential component of delivering the energy transition and it brings with it a wide array of new challenges, such as reduced inertia, but it also offers new opportunities. This TB has found that it is important to distinguish between those challenges related directly to the loss of inertia and those challenges that emerge because of the more general reduction in synchronous generation. This would be recommended practice whenever considering new challenges posed by the energy transition as different mitigation measures may be available for each challenge and when developing solutions, it will likely be most efficient to consciously group challenges that can be mitigated in the same way, rather than simply seeking to develop a like for like replacement for the lost synchronous generation e.g., stability products that supplements inertia as well as grid strength.
Many utilities are performing detailed studies of their high renewable future to assess their future inertia and frequency containment reserve needs and this TB has found that many of these studies have identified that the system is secure for future operation. This does not indicate a lack of issues, rather that utilities have already developed appropriate mitigations, which primarily consist of an appropriate inertia floor/RoCoF limit and an FFR service. In addition, many of these studies incorporate certain special schemes to manage specific contingencies. Key takeaways from these studies are that fast-responding reserve can reduce minimum inertia requirements and begins to dominate the frequency response in low inertia systems but this may result in negative impacts as well, e.g., frequency overshoots and oscillations. Another feature of these studies is the emergence of potential concerns around regional RoCoF in interconnected systems – where large disturbances in regions with very high penetrations of IBR may experience much higher RoCoFs than the rest of the system. This form of regional inertia may bear further investigation in the future especially in light of system split concerns.
Whilst many systems have identified inertia floors from these studies these tend not to be binding for current operation of all but the smaller islanded systems or systems with specific RoCoF constraints from loss of mains relays. Therefore, at this time, very few systems place direct value on inertia as the inertia provided naturally by their energy/frequency reserve market solutions is currently sufficient. However, with the growing relevance of low inertia issues and the potential future monetisation of inertia, greater focus has been placed upon the estimation and forecast of system inertia.
The TB has reported that a great many technologies can already provide the required capabilities for FFR and inertia, and a diverse range of regulatory and market mechanisms are being deployed to secure the required capability. Furthermore, grid forming converters may offer an opportunity to secure virtual inertia from IBRs but grid forming controls are still an active area of research and development.