Overhead Superconducting Power Transmission
Electricity is basic to modern life. Clean generation and storage of electrical power are the subject of much conversation, but transmission, that power’s long-distance transport, is less considered.
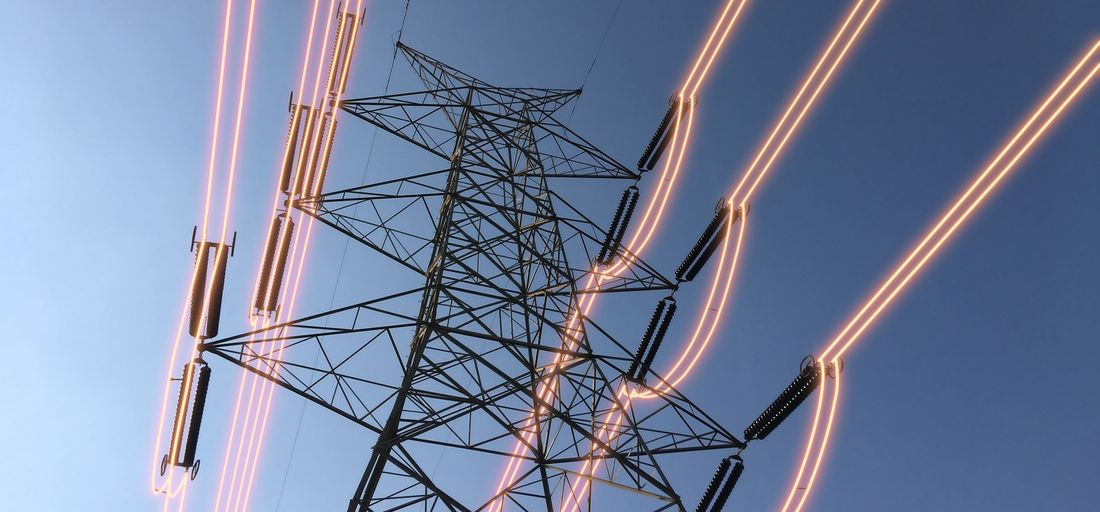
By Nathaniel Shields, Steve Ashworth, Tim Heidel, Kyle Thomas, Franco Moriconi
We need more transmission
Modification of the United States’ grid to improve its resilience and meet emissions targets, increases in electricity consumption, and the retirement of existing infrastructure all dictate a substantial expansion of transmission capacity. Research studies [2-6] indicate that a doubling or tripling of global transmission capacity is necessary to meet emissions targets. Princeton University researchers found a 1% increase in the rate of transmission installation above historical averages would reduce the US’s 2030 emissions by nearly 20% [7]. New solar and wind plants need be wired to all those electric cars and industrial facilities. New transmission infrastructure, by improving reliability during ordinary operation and resilience amid natural calamities, often reduces system cost [9-12], particularly as a changing climate increases the frequency of such events [16]. Global electricity demand, long correlate with per-capita GDP and standards of living [0], continues to climb by 1%-2% per year [1]. And existing infrastructure is due for replacement. American Electric Power, the US’s largest transmission owner, reckons 30% of its transmission assets must be rebuilt in the next decade [8]. But building new transmission is hard.
Finding new corridors, strips of land on which to build transmission lines, or expanding existing corridors can prove impossible. Nimbyism often reigns supreme. Black and Veatch, an engineering consultancy, reports that 40% of transmission industry professionals consider siting and environmental permitting the largest barriers to transmission expansion [13].
The market is ripe for a technology enabling the transmission of more power in existing corridors.
Superconductors can help
Superconductors will transform transmission. The cost and complexity of superconducting transmission systems will limit their initial use to projects requiring exceptional transmission capacity density, but as perceived risk and fundamental costs fall, use will become widespread. As the performance of superconductors differs from conductors traditionally used in transmission, they will motivate innovations across the power system’s infrastructure.
Superconductors are materials that exhibit zero electrical resistance, allowing the transmission of orders of magnitude more electricity (for fixed cross-sectional area) than conventional conductors like copper or aluminum. To understand why superconductors might be useful in the grid, analogies to plumbing can be illustrative. Electricity is the movement of charges, typically electrons. Ampacity corresponds to the amount of electricity a transmission line can transport (electrons per second), analogous to the diameter of the pipe, while voltage is analogous to the pressure of water in the pipe (energy per electron). The total power transmitted by a conductor is the product of its current and voltage. In traditional conductors, power loss decreases (quadratically) as current decreases or voltage increases. Low electrical losses are essential for economic long-distance electricity transmission because losses are power and profit wasted, but also because losses are realized as heat, which causes the transmission lines to expand and sag closer to the ground. Hence, high voltage is required—100 kV to 1000 kV is typical of transmission lines today. Air is used to insulate overhead transmission lines. As line voltage increases, the gap of air required to insulate the line, preventing sparking to the ground, increases—just as a larger water pressure necessitates thicker pipes. High voltage overhead lines are therefore suspended high in the air on imposing towers. Superconductors are different. The losses in superconductors are independent of line voltage, and superconductors can carry substantially more current than conventional conductors. Superconductors are like larger diameter, smoother pipes. Superconducting lines can therefore transmit the same power as conventional overhead lines at lower voltages but higher currents, yielding the associated decreases in infrastructure scale.
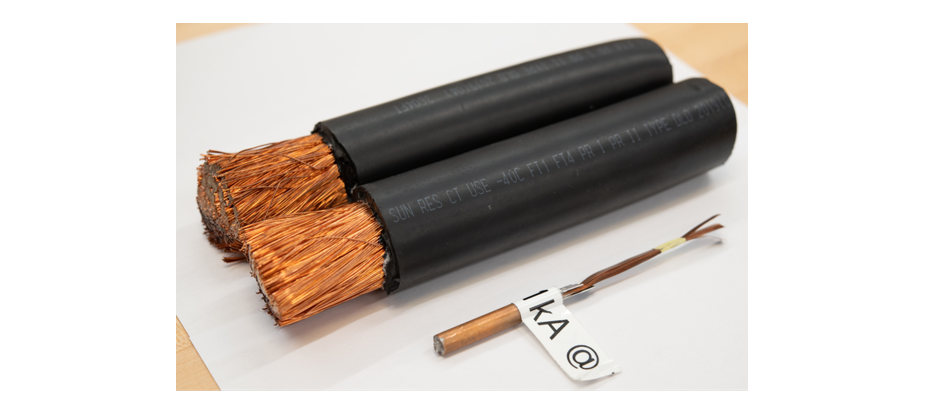
Figure 1 - Cross-sectional comparison of the superconducting tapes and conventional conductor required to transmit 1 kA. The superconducting layer in each tape is only one or two hundred-thousandths of a meter thick, limited by the challenge of aligning the constituent superconducting crystals. Future increases in superconductor thickness could massively increase current capacity. A cylindrical copper termination caps the superconducting tapes, easing current injection
Innovations in superconductors for the grid are coming. New overhead superconducting lines will be designed to integrate into the grid of today, allowing enormous performance improvements over existing conductors by transmitting a few times more current than is typical. VEIR, a startup, is producing overhead superconducting lines that thereby enable about five to ten times capacity expansion of existing corridors, depending on whether AC (alternating current) or DC (direct current) lines are installed. An existing 115 kV, 180 MW overhead line could be replaced with an overhead 115 kV, 1000 MW superconducting line. Alternatively, that 180 MW might be sited in a new, smaller corridor using a 35 kV, 180 MW superconducting line. Allowing for the evolution of substation infrastructure, planning tools, and regulation, superconducting lines might eventually operate at ampacities twenty times greater than existing transmission lines. The increased performance of superconducting lines, however, comes with additional complexity.
Superconducting transmission lines have their complications. Only aligned crystals (and the occasional narrow gap) exhibit superconductivity, and only then at very low temperatures. Processes capable of producing long lengths of aligned crystals, inherited from the semiconductor (computer chip) industry, yield thin films one or two hundred-thousandths of a meter thick, which are typically layered on a metal substrate, forming flexible tapes. A tape’s current capacity is limited to that of its worst-manufactured subsection. As tape length increases, the likelihood a section forms poorly increases; longer tape lengths have lower yields and higher per-length costs. Long tapes are desirable for long-distance transmission lines. The tapes are relatively fragile, complicating their fabrication into cables. More challenging, however, are the operational temperature requirements of even the present generation of superconductors. Modern superconductors become (very) resistive at temperatures above about 100 K (-173 °C; -280 °F). A simple way to meet such temperature requirements is to submerge the superconductor in a flow of cryogen. Liquid nitrogen, inert and with a boiling temperature of approximately 77 K at sea level, is a common choice.
The nearly two dozen grid-connected superconducting transmission projects constructed in the last three decades have used closed-loop liquid nitrogen cooling systems, wherein the thermal mass (2 kJ/kg-K) of nitrogen provides cooling power. The recooling plants for such systems are large, complex, and expensive, limiting widespread adoption of superconducting transmission lines. Alternative cooling systems that exploit liquid nitrogen’s substantial heat of evaporation (204 kJ/kg) might fare better. For example, a portion of the liquid nitrogen flowing over the superconducting cable can be periodically bled into vats to evaporate, cooling the vat to nitrogen’s local boiling temperature. Most of the flow, unbled, can then be recooled as it continues through a heat exchanger in the vat. Progress has been made toward realizing such systems; VEIR has constructed and tested a 100 ft evaporatively cooled superconducting power transmission line with two periodic cooling elements at 4 kA.
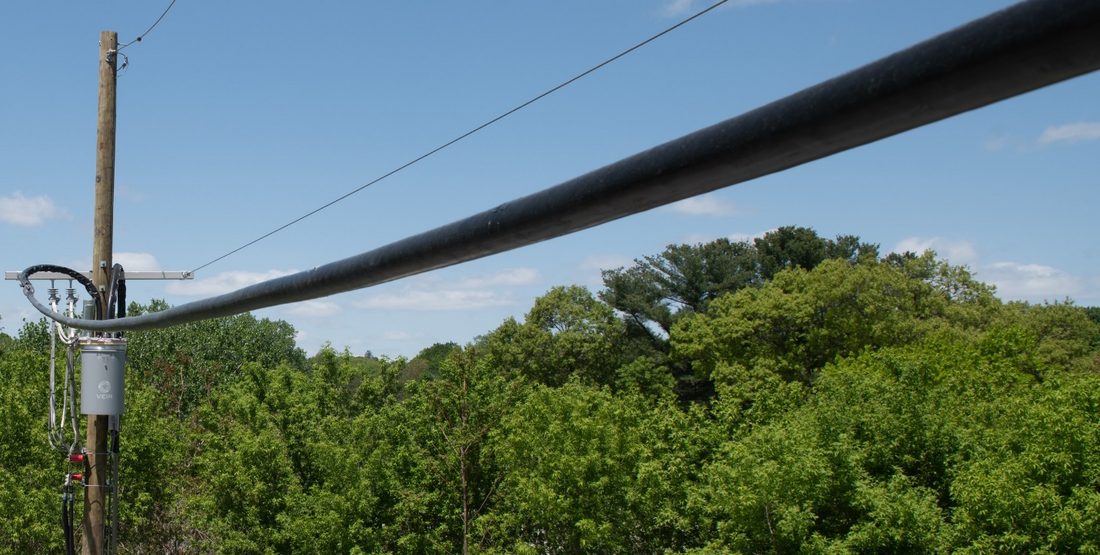
Figure 2 - VEIR’s first outdoor superconducting power transmission line, capable of transmitting 4 kA in a single-phase overhead conductor
Evaporated nitrogen is lost from the system, so additional liquid nitrogen must be supplied. Liquid nitrogen supply chains are widespread and mature, and given an appropriate power supply, liquid nitrogen is easily produced from air (which is 78% nitrogen). Plants for liquid nitrogen resupply and repressurization can be much sparser than the periodic cooling elements, up to about 100 km apart, reducing footprint and cost as compared to superconducting transmission systems with closed-loop cooling. All these complications, however, add cost atop the already-pricey superconductor. Superconducting lines are economically competitive with conventional transmission lines on a per-MW-km basis, but their perceived additional technological risk will likely lead to initial implementation in projects for which capacity density is at premium. As perceived technical risk decreases, the cost of superconducting material falls, and firms begin to scale superconducting transmission line production, superconducting transmission lines’ advantage will increase, stimulating innovations in associated power infrastructure, allowing greater ampacity and further performance advantage.
Penetration of superconducting transmission lines will motivate innovation across the power sector. Realizing ampacities greater than five times those of conventional conductors will require new substation equipment: high-ampacity switchgear, relays, etc. Superconducting lines have negligible resistance and can be designed with impedances similar to or much lower than traditional lines. When subjected to large currents, superconductors return to their normal (resistive) state, naturally limiting their exposure to faults. And superconductors have no DC loss, apart from the energy required by liquid nitrogen infrastructure. Such differences will enable a reimagination of our grid’s architecture.
We need more transmission. Superconductors can help by transmitting more power in existing corridors. Superconducting transmission lines are more complex than traditional transmission lines but offer such tremendous capacity advantages as to be cost-competitive today. Superconductors will enable a more efficient, resilient, clean, and compact grid.
Author’s Contributions: All authors are of VEIR Inc. NS wrote the article. SA developed many of the technologies described. TH leads VEIR and KT its product. TH and KT edited.
References
- Rao, N. D., & Pachauri, S. (2017). Energy access and living standards: Some observations on recent trends. Environmental Research Letters, 12(2). https://doi.org/10.1088/1748-9326/aa5b0d
- Ritchie, H., Roser, M., & Rosado, P. (2022). Energy. OurWorldInData.org. https://ourworldindata.org/energy
- Mai, T., et al. (2012). Renewable electricity futures study, volume 1: Exploration of high-penetration renewable electricity futures. National Renewable Energy Laboratory, 2(9), 2-10, and 2-11.
- Brown, P. R., & Botterud, A. (2020). The value of inter-regional coordination and transmission in decarbonizing the US electricity system. Joule, 5, 115-134. https://doi.org/10.1016/j.joule.2020.11.011
- Larson, E., et al. (2021). Net zero America: Potential pathways, infrastructure, and impacts. Final Report, Princeton University, 112-140.
- Office of Energy Efficiency & Renewable Energy. (2021). Solar futures study. U.S. Department of Energy. https://www.energy.gov/sites/default/files/2021-09/Solar%20Futures%20Study_0.pdf
- P. Denholm et al., “Examining Supply-Side Options to Achieve 100% Clean Electricity by 2035,” National Renewable Energy Laboratory (2022), pp. xi, 43-50.
- Jenkins, J.D., Farbes, J., Jones, R., Patankar, N., Schivley, G., “Electricity Transmission is Key to Unlock the Full Potential of the Inflation Reduction Act,” REPEAT Project, Princeton, NJ, September 2022.
- Reese, D., & Scott, T. (2022). American Electric Power: UBS Winter Conference, 46.
- Bloom, A., et al. (2022). The value of increased HVDC capacity between eastern and western U.S. grids: The interconnections seam study. IEEE Transactions on Power Systems, 37, 1760-1769.
- American Council on Renewable Energy (“ACORE”) and General Electric International, Inc. (2021). Potential customer benefits of interregional transmission.
- Pfeifenberger, J., et al. (2021). Transmission planning for the 21st century: Proven practices that increase value and reduce costs. The Brattle Group and Grid Strategies LLC.
- Goggin, M. (2021). Transmission makes the power system resilient to extreme weather. ACORE and Grid Strategies LLC.
- Black & Veatch. (2020, June). Strategic Directions: Electric Report. https://bv.com/2020-electric-sdr
- Hess, D., et al. (2022). End of the line: environmental justice, energy justice, and opposition to power lines. Environmental Politics, 4, 663-683.
- Cohen, J. J., et al. (2016). An Empirical Analysis of Local Opposition to New Transmission Lines Across the EU-27. The Energy Journal, 37, 59-82.
- Field, C. B., Barros, V., Stocker, T. F., Dahe, Q., Jon Dokken, D., Ebi, K. L., Mastrandrea, M. D., Mach, K. J., Plattner, G. K., Allen, S. K., Tignor, M., & Midgley, P. M. (2012). Managing the risks of extreme events and disasters to advance climate change adaptation: Special report of the Intergovernmental Panel on Climate Change (9781107025066).
Banner & thumbnail credit : jitendrajadhav on iStock