Hybrid systems for Off-Grid Power Supply
Access to electricity is still lacking for some 1.1 billion people in developing countries, many of them living in rural areas. With “electrification business-as-usual” as many as 1.4 billion people will still lack access to electricity in 2030, the UN target year for universal access to modern energy services, including electricity. Further, there are many thousands of off-grid power systems in the world – for example remote mines, natural islands, and remote communities. Many generate electricity from fossil fuels, resulting in very high electricity costs together with unwanted side effects of greenhouse gas (GHG) emissions, noise and fuel spills. The security of supply of the fuel is an issue for remote areas or in case of major events, such as extreme weather conditions.
Convenor
(DE)
B. BUCHHOLZ
Secretary
(RU)
P. CHUSOVITIN
H. BITARAF (US), M. ROSS (CA), J. GLASSMIRE (US), H. FARAG (CA), J.C. SMITH (US), R. SEETHAPATHY (CA), M. ALBU (RO), K. ASH (AU), M. BRUCOLI (UK), P.H.R.P. GAMA (BR), T. FUNABASHI (JP), P. KOTSAMPOPOULOS (GR), K. YUKITA (JP), S. TSELEPIS (GR), A. ZOMERS† (NL)
Corresponding Members: H. FARHANGI (CA), E. SILVA (BR), N. LUIB (DE), J. ADERALDO LOPES (BR), C. MARNAY (US), P. LOPES CAVALCANTE (BR)
To meet decarbonization targets of the Paris Agreement while providing universal access to electricity calls for renewable energy solutions. It is a key strategic goal of CIGRE to provide electricity for all in a sustainable way.
Hybrid systems are a combination of various generators, such as conventional generation and renewable energy (RE), and often incorporate energy storage. In off-grid areas, hybrid systems are a promising step in decarbonization. Small amounts of renewables can be added to existing diesel or gas gensets systems without any additional control or network stabilization technologies. When the contribution of renewables reaches a certain limit, a control system is needed to stabilize power quality. When adding high contributions of renewables, the addition of storage is needed to stabilize the power system. An advanced microgrid control system and an adequate grid-forming power conversion system together can stabilize the grid even if 100% renewable penetration is reached, and ensure stable, sufficient power quality at all times. Increasingly lower cost renewable generation and energy storage, together with modern controls and power conversion systems, mean that today economics and environmental considerations frequently align. In many cases, the best business case is to add significant amounts of storage and renewables to reduce emissions from conventional generation.
The Technical Brochure shares experiences on techno-economic pre-feasibility studies. Further, the study shows the necessity of stability studies and shares examples for hybrid systems for off-grid power supply. It further presents experience from systems that have been successfully in operation for several years. It is a proof of the concept that hybrid systems for off-grid power supply are already state-of-the-art and that high shares of renewable energy resources can be achieved while keeping power quality within the prescribed limits.
As an outlook, modelling and stability studies become more and more important. A benchmark model for hybrid off-grid systems and microgrids would be useful in addition to the already existing CIGRE Benchmark. This will support informed decisions by valid comparison of different control algorithms and power conversion systems e.g. during tendering phases of projects.
Goal of the Working Group
UN Sustainable Development Goal Number 7 calls for universal access to sustainable energy by 2030. Initiatives such as “Sustainable Energy for All” (SE4ALL) are a global platform that empowers leaders to connect stakeholders and unlock finance to achieve universal access to sustainable energy. The purpose of this Technical Brochure is to bridge knowledge gaps on hybrid systems for electricity supplies in off-grid and remote areas. It serves as a guide for governments, entrepreneurs, technical experts and financing organizations.
Description of the brochure
The work includes five chapters and an appendix for the example case descriptions.
Introduction
Hybrid systems use a mixture of distributed energy resources, including solar, wind, hydro, biofuels and fossil fuels, combined with energy storage and controlled by an energy management system. By presenting experiences related to existing hybrid systems for off-grid power supply for different applications, this study can offer broad support for the preparation of installations for remote power supply, in particular those using renewable energy.
When considering bringing modern energy services to an off-grid area, three primary modalities are typically requested:
- Extension from an existing utility grid, although this is challenged by geography and capacity constraints on the existing utility grid.
- Microgrids, which can be powered by fossil-fuel, 100% renewables, or a hybrid combination of these [1]. Microgrids can run islanded or grid connected. In off-grid areas, these are sometimes referred to as mini-grids.
- Small independent systems, whether solar home systems for the residential sector or productive PV for powering businesses.
Focus is on hybrid systems for off-grid areas. In this context, we refer to microgrids as a common technology for off-grid power supply. They may also be deployed as part of a broader grid edge approach, which is a distributed approach in contacts to traditional centralized planning and operation.
The need for hybrid systems in off-grid areas
This chapter is targeted mainly towards financial institutions and management in distribution utilities and mining. The chapter discusses both technical and economic challenges for hybrid off-grid systems. It suggests a business case methodology that shows under which assumptions and in which scenarios a hybrid system provides a solid payback time, internal rate of return and net-present cost.
Appropriate modelling tools are critical to ensure the success of a particular hybrid project. Initially, a project will need to establish what is technically possible and economically viable, while complying with social and environmental needs. As the UN Energy Access Practitioner Network notes, “understanding how to best combine traditionally generated and renewable power, storage, and load management for the optimal site-specific installation, at the lowest cost, is the key for deploying efficient mini-grid solutions.” [2] This will ensure that the project will likely meet the overarching goals and will improve the likelihood of funding from investors. Subsequently, additional modelling will likely be needed to finalize the design and technical assumptions.
The Technical Brochure presents an initial techno-economic evaluation for a hybrid power system to improve an island utility. The modelled island has an average 11.2 MW load, with occasional load peaks up to 15 MW. The utility may choose to make a series of staged investments, or incremental hybridization investments, to stage capital investments and follow a less sudden energy transformation. Initially, an investment in a BESS is recommended to improve network stability and improve power quality, ensuring that subsequent renewable investments can be more optimally used with less curtailment. After an initial BESS investment, significant PV and additional BESS may be installed to reduce costs and increase renewable contribution. Finally, more solar PV and BESS can be installed to further reduce costs and increase renewable contribution while minimizing overall costs.
The evaluation in the brochure considers four scenarios. Figure 1 shows the “Medium renewable” and “High renewable” scenarios as an example. Focus has been put on investment options that provide a viable payback, adequate internal rate of return (IRR) and suitable total lifecycle cost. In this example, the “high renewable” scenario represents the generation mix with the lowest levelized cost of energy (LCOE). Projects with lower carbon emissions and increased renewable usage are possible, and a similar approach may be used to screen for the lowest cost options that meet those social and environmental needs. The selected incremental investments are summarized in Table 1 [3].
With decreasing cost of PV, wind and storage and increasing robustness of control algorithms, hybrid systems have become an economically viable option for off-grid power supply. It will be important to raise awareness of this proven technology in order to deploy them more rapidly in remote sites.
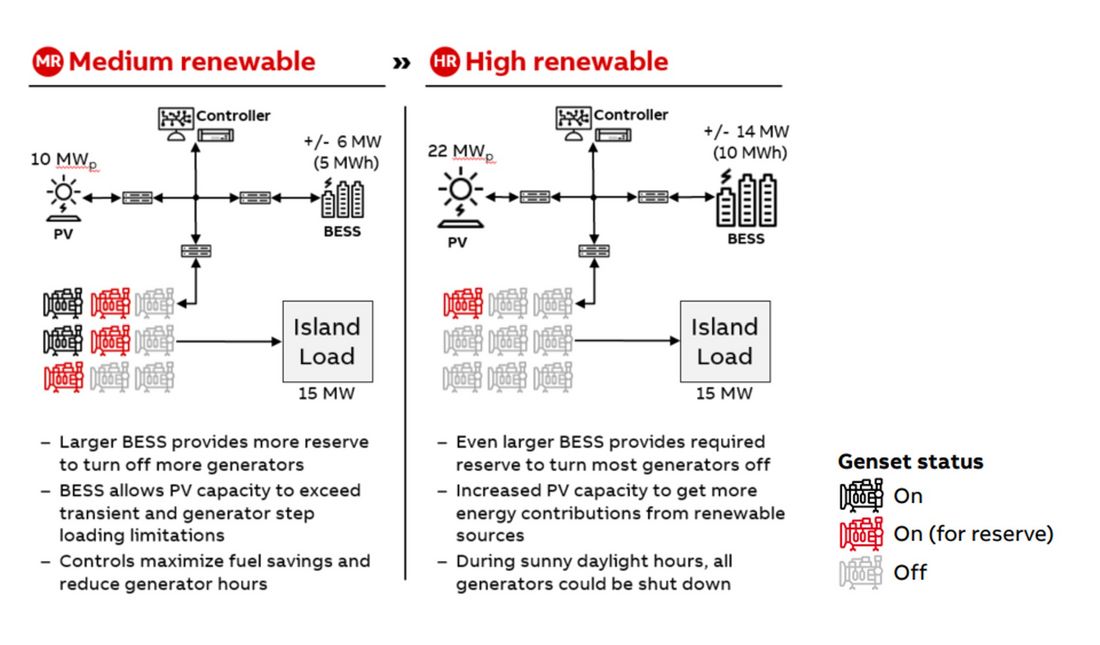
Figure 1 - Extract of scenarios for a path to high renewable shares in off-grid power supply
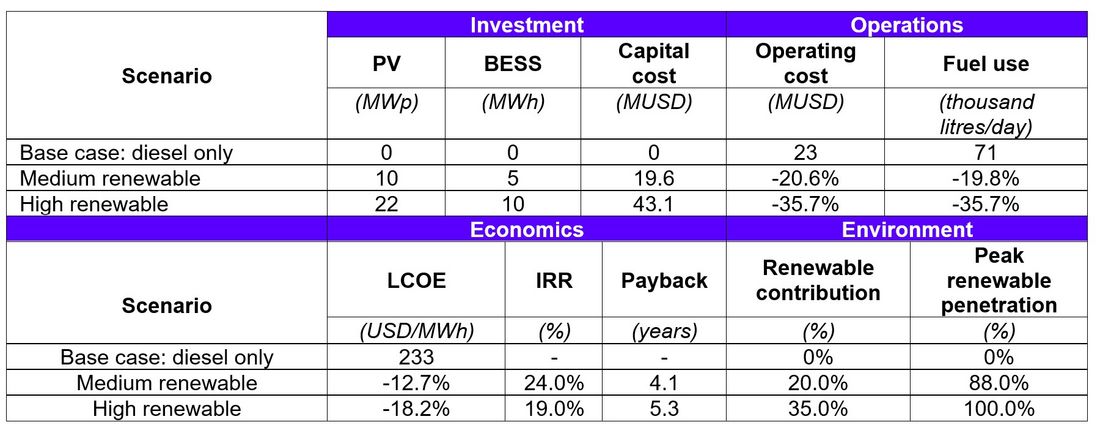
Table 1 - Summary of operational benefits and economics for an island utility making hybrid investments
Planning hybrid off-grid systems with high renewables penetration
Sizing and application engineering request a set of studies – starting with techno-economic feasibility, then continuing with stability studies for the power system in the adequate simulation tools. The chapter shows examples of off-grid hybrid systems engineering for selected applications in mining, and examines more thoroughly dynamic stability studies for islands and remote communities.
A hybrid system can include different distributed energy resources such as generation based on Diesel or gas, Photovoltaic (PV) systems, Wind turbines or hydropower, as well as energy storage systems (ESS), and loads under one control system.
Off-grid or stand-alone systems are different from centralized power systems in terms of capacity and voltage level. The capacity of off-grid systems is less than several hundred megawatts (MW), while centralized grids are in the range of gigawatts (GW). The voltage level at off-grid systems varies by application and could be in the range of medium and low voltage levels, while centralized grids include transmission and high voltage levels. Off-grid systems are not connected to neighboring grids to balance the mismatch between generation and load, while centralized grids are connected to their neighbors via tie lines. Off-grid systems have low inertia and have frequency fluctuations, while centralized grids are stable with high inertia.
The Technical Brochure shows key parameters needed for simulating how generators, storage and loads operate in an off-grid power system with a focus on mines, islands and remote communities. Among others, it provides an example for simulating a response to step changes. The results of the step-change load scenarios for an island are summarized in Table 2. The maximum frequency deviation for all step changes has been reduced significantly by the introduction of the BESS and microgrid control system into the island system. The prompt response from the energy storage avoids the need to shed load. The response time of energy storage is calculated based on the time it takes the BESS to reach 90% of the step change. The BESS can reach 90% of the step-change in six milliseconds. This time duration is measured from the time the step-change is applied to the time the BESS reached 90% of the change. The duration also includes the delay regarding sensing and communication, summarized as PLC (power line carrier) update time, considered to be 0.5 seconds. This prompt response provides the frequency regulation and ramp rate control for the proposed wind installations and crane operation.
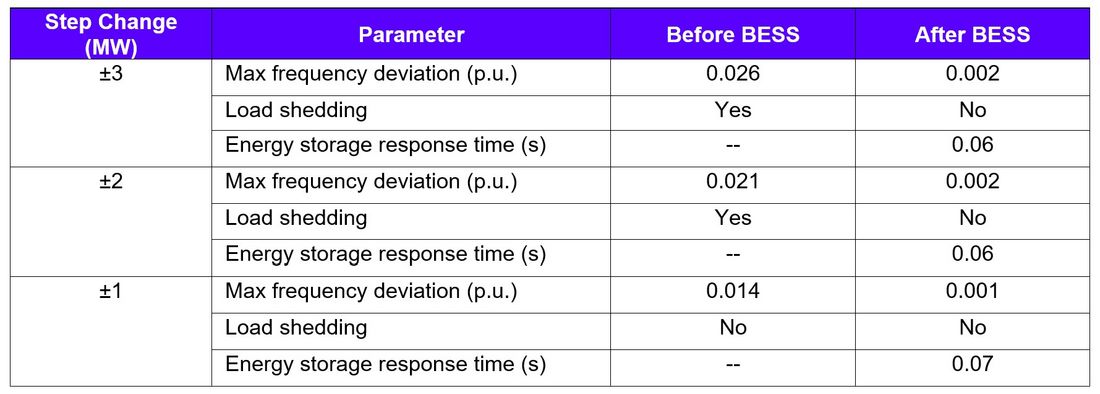
Table 2 - Island utility response to load step changes before and after BESS integration
Another example in the brochure shows how a grid impact study (or power system impact study) is used to assess the impacts of integrating renewable generation or distributed energy resources in remote communities for the creation of an off-grid hybrid system. Several studies can be performed to determine not only the specific bottleneck that can prevent a higher integration but also the specific limit of integration that allows for a responsible integration [4].
The adequacy of the system is determined through a quasi-static time series analysis to evaluate the steady-state energy balance of the system [5]. The purpose of these studies is to ensure that power balance can be met for all times during the projected lifespan of the hybrid system equipment and all possible operating conditions. Reserve margins, system voltage profiles, and operating setpoints of the generators and other controllable distributed energy resources can be evaluated to ensure that no operational thresholds are exceeded. As a general principle when integrating new resources into an existing off-grid system, it is favourable to connect at the existing diesel plant bus since:
- the diesel generator can regulate the voltage at the point of common coupling without requiring ancillary service support from the additional resource, and
- the standard downstream power flow of the system is maintained, thus abiding by the predesigned protection coordination.
The resiliency of the system is assessed through a small signal stability analysis. Since the load profile in a remote community is constantly fluctuating, small signal stability analysis will determine whether the primary controls in the system can ensure stability under small perturbations. The dynamic operation of the power system equipment and the primary controls are linearized around a quasi-static steady-state operating point, and the eigenvalues of the linearized system will mathematically provide insight into the system’s stability margins. If the eigenvalues are all in the open left-hand plane, the operating point is stable for small perturbations around that point. This must be done for all balanced points in the system identified in the quasi-static time series analysis.
Security is assessed by identifying the largest acceptable disturbance to which the hybrid system can be subjected (often it is the direct connection or disconnection of the renewable generator) and simulating the response of the system and assessing if any equipment or system thresholds are exceeded. This continuous-time analysis must reflect the worst-case scenario for a large disturbance, and appropriate models of the equipment and controls found in the off-grid system must accurately reflect their operating dynamics. An example result of this study is shown in Figure 2, which shows the frequency and voltage of the plant bus after a loss of 256 kW of solar generation in the Old Crow off-grid system [6]. Safety is evaluated through the analysis of the protection system. Furthermore, reverse power flow and protection coordination must be evaluated.
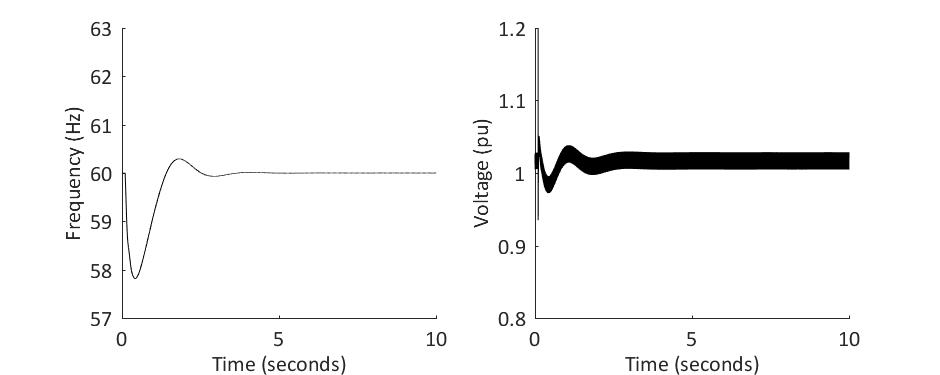
Figure 2 - Plant bus dynamic frequency and voltage after an instantaneous disconnection of 256 kW of solar photovoltaic generation
Examples for hybrid systems for off-grid power supply
This chapter presents an overview of 26 worldwide case studies/ installed systems for off-grid hybrid systems from all over the world. It shows 4 remote mines, 16 islands, and 6 remote communities. The cases are evaluated regarding key technical data, operation, economics, and decarbonization impact. The case studies offer very interesting insights into power balancing and voltage control despite the power system architecture being very varied amongst themselves. Analysis of the collected case studies reveals some best practices for renewable energy generation maximization and reliability improvement methods for off-grid power systems. For cases where 100% renewable systems (i.e., wind and/or solar) are to be deployed, using energy storage systems (ESS) is a must. Moreover, for such RE and ESS systems, the storage inverter must be capable of operating in grid forming mode. For systems with adequate fossil fuel generation, the application of energy storage can substantially reduce RE generation curtailment. Technical details of the systems include generation, demand, distribution systems, control and communication. Further details of the cases are listed in Appendix C.
For each segment Mines, Islands and Remote communities, the aspects of technology, operation, economics and decarbonization are described.
Table 3 shows a summary of cases. Classification of off-grid systems follows typical applications:
- Mines and other Industrial/commercial;
- Islands;
- Remote communities.
There are four case studies for mines, Islands have 62% of all case studies, which is natural since such systems have more reasons to increase renewables penetration. It is expensive both to connect islands to the grid and to deliver fuel. Islanders consume more energy per capita than comparable consumers on the mainland.
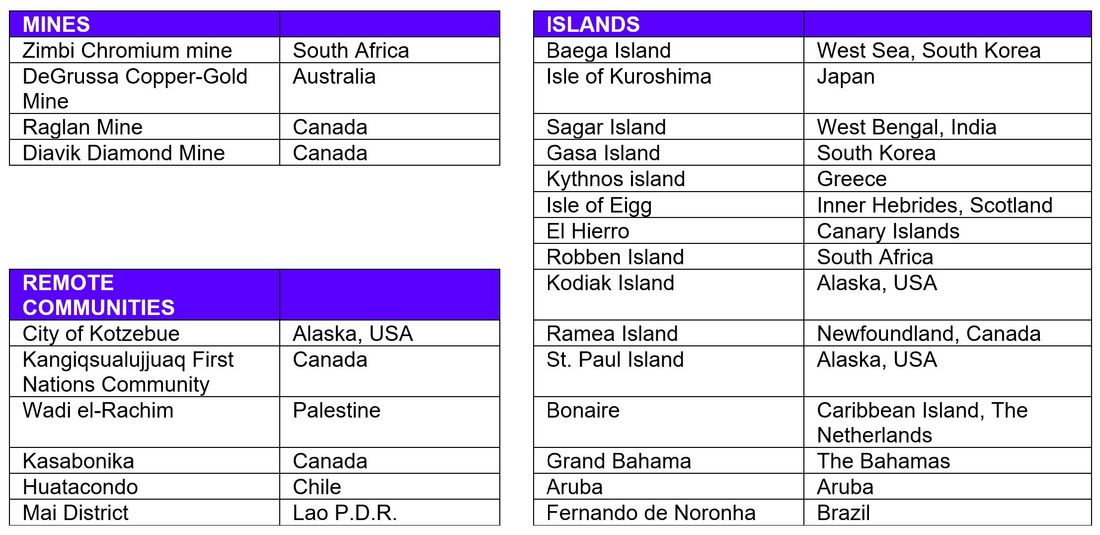
Table 3 - Overview of cases described in the Technical Brochure
Various case studies offer very interesting insights to power balancing and voltage control despite the fact that the power system architectures are very varied. Analysis of the collected case studies reveals some best practices for RE generation maximization and reliability improvement methods for off-grid power systems. For cases where 100% renewable systems (i.e., wind and/or solar) are to be deployed, using energy storage is a pre-requisite if there is no renewable base generation unit available, such as geothermal, biomass or hydro power. Moreover, for the systems based on variable renewable sources and electrical energy storage, the storage inverter must be capable of operating in grid-forming mode. For systems with adequate fossil fuel generation, application of ESS storage can substantially reduce RE generation curtailment.
It is expected that off-grid power systems of the future will deploy more hybrid architecture (using various ESS systems) to increase the RE generation to almost 100%, both because of decarbonization of generation, as well as for economic reasons as this reduces consumption of fuel delivered at high cost. To achieve all this, the use of fast-acting, advanced control algorithms and features in both power balancing (dynamic power control) and voltage/var control will be necessary in future hybrid controlled power systems.
Conclusions and key learnings
This Technical Brochure is a proof of the concept that hybrid systems for off-grid power supply are already state-of-the-art and that high shares of renewable energy resources can be achieved while keeping power quality within the prescribed limits under different operating conditions. As modelling and stability studies become more and more important, a benchmark model for hybrid off-grid systems and microgrids would be useful in addition to the already existing CIGRE Benchmark. This will support informed decisions by valid comparison of different control algorithms and power conversion systems e.g. during tendering phases of projects.
- [1] Hatziargyriou, Nikos, ed. Microgrids: architectures and control. John Wiley & Sons, 2014
- [2] Glassmire, John, “Using Software to Make a Compelling Business Case for Mini-grid Deployment”, United Nations Foundation, Energy Access Practitioner Network, June 2019.
- [3] ABB, “Increasing renewable contributions in island utility grids”, July 16, 2019
- [4] ABB, “Increasing renewable contributions in island utility grids”, July 16, 2019
- [5] Zrum, J. Sumanik, S., Ross, M., “Power System Impact Study Study Methodology”, Northern Energy Innovation, Yukon Research Centre, Yukon College. Mar. 31, 2018.
- [6] Ross, M., Zrum, J., Bos-Jabbar, T., Bulut, S., Dohring, T., Favreau, G., Hynes, A., Rahman, T., Ross, J., Sumanik, S., Thompson, S., Tutton, R., “Grid Impact Study for Old Crow Solar Project”, Northern Energy Innovation, Yukon Research Centre, Yukon College. Feb. 9, 2018.