The Condition of Solid Transformer Insulation at End-of-Life
Per definition and in practical terms, the "end of life" (EoL) of an apparatus is the time when the apparatus is taken out of service for scrapping. In the normal and by far most frequent case, this moment is a deliberate, precautional measure by the operator/owner – and mainly a decision taken due to age (condition) of the device and / or to economic and risk considerations. In very rare cases, the scrapping follows a catastrophic failure, which made the apparatus irreparable, or alternatively too expensive to repair, also taking into account its actual (age) condition. In this article, the EoL term is used as the age (condition) of the transformer, more precisely to when it is physically prone to fail at any moment, thus is no longer safe to continue operation due to degraded solid, cellulosic insulation.
By Christoph Krause (CH) convener, Alfonso de Pablo (ES), François Devaux (FR), Hongzhi Ding (GB), Victor Katshuna (NA), Jelena Lukic (RS), Lena Melzer (SE), Satoru Miyazaki (JP), Mike Munro (GB), Anabela Peixoto (PT), Mario Scala (AT), David Walker (GB)
Introduction
The present report details the issues around the physical EoL parameters, discusses unknown correlations of aging records with aging status of solid insulation, and unveils and describes related gaps in knowledge. Uncertainties are generated and associated to monitoring data, oil sampling challenges with temperature and related water, acids, furanic compounds partition, mainly 2FAL (2‑Furfural) occurrence dependence on paper thermal upgrading and loss due to oil change and/or treatments, etc. Answers to a questionnaire from 44 utilities give insight to today’s EoL assessment practices worldwide. Elaborating on the physical properties of aging cellulosic insulation along with the postulation of safe operation, work is proposed to be undertaken in order to answer the questions transformer owners have on how to handle their aging transformer fleet from their practical "end-of-life" perspective.
Aging of transformer insulation
Thermal stresses [1], in combination with oxygen (always present dissolved in the oil) and in the presence of copper catalyst lead to degradation of transformer insulation, both liquid and solid, and subsequent formation of by-products [1-4]. The presence of furans in transformers that failed due to overheating was first described by Peter Burton in 1982 [5]. As early as 1985, Shroff and Stannett found a logarithmic relationship between furfural content and the DP of paper [6]. Now, the most widely used interpretation models to correlate furans content with DP are those of Chendong [7] and Stebbins [8]. It is noteworthy that these two methods are based on the statistical evaluation of transformers in service. To overcome this limitation, a different (de Pablo) model was developed, [9]. The above mentioned models can give false or imprecise estimations of the DP because of the following constraints, [10]: ignorance of the actual amount of paper that is being degraded at a given time; ignorance of transformer’s thermal profile; effect of oil treatments on furfural concentration; thermally upgraded paper does not generate sufficient 2FAL; effects of oil condition (water/ and acidity) on furans production and dissolution in the oil; dependence of furan measurement on temperature due to partition (transients) with cellulose. The precision can be improved by introducing the temperature distribution in the insulation, e.g. [11], aging models and aging curves that take the water content in the paper into account, which modify the paper degradation rate and the solubility of aging products (markers) in the oil, [12-13].
Additional aging effects impacting lifetime are (a) mechanical shock(s) and vibration during transportation and operation (cumulation of high and low current short-circuit events) [14]); (b) on-site installation shortcomings and mistakes; (c) lightning and switching impulse(-s) despite arrestors being installed [15]; (d) (“supra”-harmonics, repeated superimposed high-frequency stress (photo-voltaic, wind generation specifics, industrial); (e) temporary overload(s), including bubbling effects [16].
Lifetime of transformers and End of Life considerations
EoL of a transformer is reached when the transformer is not able to perform or function as intended, when it has ceased to be useful and is taken out of operation. The transformer EoL can be separated into technical-, economical- and strategic end of life [17]. The most common reason to take a transformer out of operation is of economical (e.g. high losses) or strategic nature (e.g. power demand, voltage level change). Technical factors, with respect to EoL of a transformer, should be separated into factors affecting the active part, the bushings, the tap changers and the cooling equipment. For each part a risk level needs to be defined, a level beyond which the transformer never should be operated.
An example of active part consideration and normal aging of paper is when the mechanical properties of paper covering conductors have changed over time so that the tensile strength and elongation of the paper will not fulfill the stresses on the windings, either during normal operation or during events causing short circuit stresses. If the paper is so brittle that it falls apart when a mechanical stress is applied, this eventually could lead to shorts in stressed areas.
Another aspect is how the paper dimensionally changes during normal aging. There are two possible scenarios, one is decrease in stress due to plastic deformation of insulation materials or relaxation in the mechanically supporting structure. Another is that the stress increases due to paper swelling, because of the water that is (a) produced during the aging reactions of the paper/and or the whole insulation system, or (b) by water ingress through leaking gaskets or through the expansion system. The moisture will be absorbed mostly in the cellulosic insulation. Dependent on the design of the clamping structure of the active part, a change in dimension of the paper can therefore either give lower or higher clamping forces. The change could cause problems for instance during short circuit stresses or other mechanical movements inside the transformer. The remaining clamping pressure is another example of a mechanically related end of life criterion [18]. Technical EoL of a transformer can also be caused by or influenced by transportation, repairs, historical short circuits, or other harsh movements.
There is no strict, clear and widely accepted definition for end of life of solid transformer insulation or a lowest acceptable parameter value. However, an often-cited end of life criteria for cellulosic based material is when the DP has reached 300-200 or less, when tensile strength is significantly less than 50 % of new paper. New material normally has a DP of about 1200-1000. The application of the criterion is, however, not clear. Whether it is valid for the most mechanically and/or electrically stressed area, whether it is valid for the warmest area or whether it is valid for the hot spots in the windings, is open for discussion. The previous edition of the IEC Loading Guide (2005) in Table 3 helped both manufacturers and asset owners to estimate how the years of operation at given hot spot temperature will affect the tensile strength and the DP of the paper, [19]. The table lists two different end points for tensile strength, either a 50 % drop in tensile strength or a 75 % drop in tensile strength, or a level of DP 200. There is a remark that these results were obtained under perfect conditions, i.e. very dry, samples under oxygen-free conditions and for thermally upgraded paper. There is also a note that the presented “lifetime” should be used as reference numbers since these numbers refer to a transformer at full load for the whole operating time which seldom is the situation in real life. It is to be emphasized that in this IEC loading guide, there is no indication of which degradation model has been used to determine the DP, nor how the significance of this figure was established [2].
Measuring DP is still the most commonly used method to assess the aging status of solid insulation: it provides a considerably good correlation with tensile strength, [20]. The DP values at selected conductor locations can vary significantly from the innermost to the outermost paper layers. The DP estimations, derived from 2FAL measurements in the commonly used equations, do not always correlate with the actual measurements. In some instances, they are a good match, but other instances can be found to deviate significantly from the actual measurements. Yet, it was repeatedly observed and reported in literature that transformers can continue operation flawlessly with significantly aged insulation (DP < 150): in those cases, the paper was neither disintegrating nor flaking off yet [21].
The alternative, which is to sample for tensile strength test, requires flat, long and wide multiple strips of paper, which cannot be found in the transformer windings nor in the transformer unless there have been some strips put there by the manufacturer for future use, with the concession that such samples are usually not experiencing the temperatures of the hot-spot. Moreover, because opening the tank for removing samples is an elaborate and delicate intervention which entails contamination hazard, it is hardly ever done.
The aging markers used to identify warming up or over-heating of cellulosic materials, such as carbon oxide, carbon dioxide, furans and alcohols, e.g. methanol and ethanol, are mostly used as markers only and for diagnosis of abnormal operation. There are no general levels accepted within the transformer community where one single marker alone would imply that the cellulosic component has come to its end of life. However, specific asset owners have data where these markers have been correlated to results from other paper tests performed on transformers taken out of operation. Such data is being used to define the asset owners’ individual end of life criteria.
Transformer Questionnaire Analysis
Participation breakdown of the questionnaire survey
The survey received a total of 48 responses from 44 utilities in 19 countries, including: Australia (3), Canada (2), China (2), France (3), Germany (3), Italy (1), Japan (4), Jordan (1), Malaysia (1), Mauritius (1), Namibia (1), Portugal (7), South Africa (3), Spain (2), Sweden (4), Switzerland (2), Thailand (2), UAE (1), UK (5).
Summary of the questionnaire survey results
- Over 93 % of the surveyed utilities (i.e. the vast majority) use oil sample test results for transformer EoL decisions. It is fair to conclude that oil testing drives the transformer EoL decisions for most of the utilities.
- The three (3) most important oil sample tests for transformer EoL decision, in order of importance, are DGA (95 %), 2FAL (84 %) and Oil Quality [3] (82 %).
- The three most popular methods for transformer EoL decision in terms of oil sample tests, in order of importance, are DGA plus 2FAL (80 %), 2FAL plus Oil Quality (70 %), and DGA plus 2FAL and Oil Quality (68 %). It is worth noting that more than 34 % of the surveyed utilities have started to use methanol/ethanol as an additional ageing marker for transformer EoL decisions.
- The three most popular oil sampling frequencies for DGA testing are 1 year (55 %), 6 months (16 %) and others (11 %).
- The three most popular oil sampling frequencies for 2FAL testing are 1 year (39 %), 2 years (14 %) and 5 years or others (13 %).
- The three most popular oil sampling frequencies for Oil Quality testing are 1 year (45 %), 2 years (11 %) and 5 years or others (7 %).
- The three most popular orders (from most to least important) for transformer EoL decisions are Oil, Bushings, Tap-changer, Tank, Other Component (22 %); Oil, Tap-changer, Bushings, Tank, Other Component (14 %); Oil, Tank, Tap-changer, Bushings, Other Component (8 %).
- The most popular method for assessing the lowest DP of solid insulation for transformer EoL decisions is calculation of DP based on 2FAL in the oil (77 %), plus other methods such as post-mortem investigations, modelling, cellulose samples inserted in the tank, and electrical condition assessment tests. It is worth noting that more than 62.5 % of the surveyed utilities use electrical condition assessment tests as an additional method for transformer EoL decisions.
- The transformer EoL decisions are not significantly influenced by the transformer size (MVA). Most of the surveyed utilities (more than 56 %) considered that transformer size does not matter or did not care, and only about 44 % of the surveyed utilities considered that bigger ones are most important.
- The transformer EoL decisions are strongly influenced by criticality, and most of the surveyed utilities (more than 77 %) consider that the most critical are the first ones to be removed from service.
- Most of the surveyed utilities (more than 58 %) consider their EoL decision-making as based on “hard-science”, but clearly there are no strict guidelines to follow – either have no written policy for transformer EoL decisions (46 %) or have no answer/information (12.5 %). Less than half of the surveyed utilities (42 %) have a written policy for transformer EoL decisions.
- At present, less than half of the surveyed utilities (42 %) consider that they are taking old transformers out of service just in time; almost half of the surveyed utilities consider that they are taking old transformers out of service either too early (23 %) or too late and taking risks (27 %); and about one third of the surveyed utilities (31 %) have suffered catastrophic or near-miss failure(s) related to transformer EoL decisions.
Questionnaire concluding remarks
DGA remains the most valuable and effective means of detecting a wide range of faults in the transformers and assisting in determining when to remove the transformer from service for repair or replacement. At present, there is no established universal DP limit for transformer EoL decisions, but the majority seem to accept DP 150‑250 as transformer EoL limit. At present, the transformer EoL decision-making does not follow strict guidelines, and there is no established ‘standard’ to follow. From the perspective of utilities, there is a real need for strict guidelines for better end-of-life determination to improve transformer EoL decision-making.
Knowledge gaps and missing tools for precise EoL assessment / determination
Regarding the EoL assessment of oil-cooled power transformers from a technical perspective, the condition of the cellulosic insulation is critical and decisive; guidance can be found in [22-25].
Parameters and conditions that determine EoL (technical end of transformer insulation life)
Summarizing from the previous chapters, there are mainly four parameters, respective conditions that may – independently from each other or in combination – determine or cause the end of a transformer in operation:
- The DP, in particular the aging status of the cellulose insulation: If the aging is advanced, this means the DP is very low, in a way that the insulating function of the paper is no longer guaranteed, and it is advisable to take the transformer out of service. Principally, there are two scenarios: (a) The paper gets brittle with age and it eventually starts to fall off (flake off) which reduces the dielectric strength, for instance from turn to turn. (b) Due to aging, the mechanical strength of the paper declines to the extent that it does no longer withstand the forces and stresses from short-circuits currents or even from operational vibrations.
- The clamping force of the windings: The static clamping force of transformer windings is subject to variation – the main contributors to that are: plastic deformation under constant pressure, moisture dynamics and change and related swelling/shrinkage effects, temperature change, elasticity change and decomposition of cellulose by aging, through-fault current events. When the short-circuit withstand strength is lower than the stresses that may occur due to short-circuits in the system, the transformer should be taken out of service, unless the major exercise of a re-clamp is feasible and economically reasonable.
- The water in the insulation system: If moisture exceeds certain levels, the insulation strength can be reduced in a way that safe operation is no longer possible. Several percent of water reduce the electric puncture and surface (creep) strength considerably. In addition, bubble formation hazard increases significantly with moisture. Depending on the circumstances, drying on site is impossible and or considered too risky due to the involved clamping pressure reduction, or when dry-out is economically pointless, then the transformer has reached its end of life although the condition of the solid insulation would still allow operation, after drying.
- Sludge formation: In rare cases, the accumulation of sludge will reduce the cooling performance of the oil flow to an extent that the transformer windings will overheat unnoticed. In such a case and if major overhaul and maintenance for clearing the plugged zones in the cooling circuit and the windings by removing the sludge is impossible or too expensive, the transformer should be taken out of service.
Issues related to the determination and assessment of EoL parameters and conditions
- The DP, in particular the aging status of the cellulose insulation. Because it would require opening the tank, it is not realistic to get paper samples from transformers in the field. Even more, the paper of the hot spot is not accessible anyway, thus the critical DP cannot be measured directly. Indirect methods (via aging markers as furanic compounds, alcohols, carbon-oxides dissolved in oil) have limitations, are not precise, e.g. interference with moisture and acidity, thermally upgrading dependent. Oil/paper mass ratio, temperature-distributions inside the insulation – varying with transformer loading, loss of aging markers from the oil (due to the evaporation, conversion into other products, oil treatments) and lack of more precise models which take the impact of water content in DP estimations into account – restricts the application of correlations from oil to insulation in an accurate way. There is not just one DP value in a transformer, but a range inside the active part (”DP-landscape”), mainly as a result of the temperature gradients and the uneven moisture distribution. Even with exact, ideal DP knowledge: correlation of DP with paper flaking-off and of DP with the ’real, required’ mechanical strength is practically unknown. The latter is a mixture of tensile, tear, burst, compression, friction and vibration resistance etc., apart from the obstacle of being design dependent. DP-simulation methods by extrapolating monitoring data have also their limitations: for one, the time zero water content and water distribution (at commissioning) are unknown. Moreover, the thermal simulations depend strongly on the hot spot calculation and on the correct positioning, mounting and functioning of the fiber optic sensors inside the windings.
- The clamping force of the windings. Today’s power transformers are in almost all cases designed and equipped with a geometrically non-variable clamping system. This entails the disadvantage of pressure change in the case the clamped windings undergo moisture change and or temperature variation, but also due to aging (cellulose decomposition). Furthermore, there are no commercially available systems for on-line clamping pressure monitoring of oil-cooled power transformers, which means that in operation the clamping condition is unknown at all times. The determination of the clamping condition by noise- or vibration measurements is not a precise science and can be misleading due to masked signals of the most critical zones. It has to be stated that even if it were possible to measure the static clamping forces of all windings in a new transformer, the clamping condition when a transformer has reached its end of life remains unknown: apart from the specific condition of the grid and the number and severity of short-circuit events that may occur, it depends strongly on the transformer design (conductors, winding build-up and clamping structure) itself, and on maintenance.
- The water in the insulation system. Whilst the moisture of oil can be determined with acceptable precision, this is not the case for water content in solid insulation. In addition, the moisture is not uniformly distributed in the active part because the partitioning (solid/liquid) is dependent on temperature, on their temperature gradients due to ambient temperature and load variation, and on the aging status of the insulation. The downside with regard to solid insulation moisture is predominantly lack of precision both concerning the absolute value and the location in the active part.
- Sludge formation. Because it is impossible to determine the presence, the exact location and the extent of sludge inside a transformer, it is practically impossible for the transformer operator to decide on the necessity of intervention.
Summary and Conclusion
Toward the end of a transformers life, safe operation is progressively impaired firstly by cellulose aging (DP reduction), but also by varying (reduced) static clamping forces of windings, by (excessive) water in the insulation system and possibly by the presence of sludge:
- cellulose aging reduces the mechanical strength of the paper, which is required for its insulating function, particularly at short circuit events with the associated dynamic forces acting on the windings.
- reduced winding clamping affects the mechanical short circuit withstand strength negatively; slack windings will only survive a fraction of current when compared with the designed short-circuit performance.
- high water content (locally > 3 %) reduces the dielectric strength, particularly the dielectric creep strength at the oil / pressboard interface.
- sludge deposit clogging the cooling ducts reduces the oil flow and thus may cause winding over-heating.
Oil flow clogging by sludge is difficult to discover, even with temperature sensors in the windings – but overall seldom an EoL reason. In contrast, the presence of water inside transformer tanks is a well-known hazard and of persistent concern. Although the exact determination of moisture amount and distribution inside the active part is still impossible today, the indirect estimation methods by oil sampling or by oil moisture sensors are accurate enough – especially using the relative moisture vapor pressure determination (“water activity”), [3] – to indicate upcoming issues due to moisture related reduced insulation strength. More awkward is the determination and the control of the static clamping of the windings: currently, there are no commercially available oil-resistant monitoring systems that can be used to observe the force variation over decades – prototypes are currently being tested.
The survey among transformer owners has revealed that the aging process of cellulose is the main focus related to end-of-life considerations. The majority of operators are assessing the insulation age indirectly by annual or biannual oil sampling: using correlation curves of markers, mainly furanic compounds, the DP of the paper is estimated. Apart from the disputed correlation curves themselves, the fact that the markers represent the integral of the aged paper entails the constraint that in the case of a relatively small strongly aged zone (e.g. due to local overheating) it is sometimes masked and thus not recognized. Yet, there were cases reported with low absolute values, but significant rise of concentration of aging markers in the oil (methanol furan derivates) indicative of localized, geometrically limited fault involving paper insulation. It is repeatedly observed that very old transformers can possess larger margins, and in harsh conditions may stand better than new transformer designs. The replacement decision may be premature in such cases.
Elaborating further on the often mentioned and used EoL DP-limit of 200:
- Paper, specifically its mechanical strength, is degrading incrementally – this means that for physical reasons there is no “sharp DP limit”, apart from the fact that the operation limit is specific to design and to operation conditions (amplitude and probability / frequency of short-circuit events).
- The operation of transformers with heavily aged insulation is principally not safe as long as the remaining capability of short-circuit withstand strength is unknown.
The main knowledge gaps for precisely and reliably assessing transformers’ end of life are:
- Clamping monitoring systems that record the winding clamping over lifetime.
- Methods for determination of the aging condition of the cellulose insulation with precision.
- The exact knowledge of the paper with lowest DP and its location in the transformer, which is more meaningful and beneficial than the currently reported number representing some kind of average, low DP.
- The design- and material-specific correlation of pressboard and paper age (DP) with mechanical resistance to short-circuit stresses, specifically the dependence on paper type, density, paper wrapping.
- Impact of operational stress (mainly overvoltage surges and the short-circuit history) of transformers on the resistance to short-circuits in the future.
- Knowledge of the correlation between the remaining clamping force, the aging condition (DP) of the cellulose insulation and the short-circuit withstand strength.
- Guidelines and recommendations of general and design-dependent operational limits (clamping force, DP), which can be used by the transformer operators for the risk assessment and management of the transformer fleet.
References
- CIGRE TB 323, "Ageing of cellulose in mineral-oil insulated transformers", Paris, October, 2007: https://e-cigre.org/publication/323-ageing-of-cellulose-in-mineral-oil-insulated-transformers
- CIGRE TB 349, "Moisture equilibrium and moisture migration within transformer insulation systems", Paris, June, 2008 : https://e-cigre.org/publication/349-moisture-equilibrium-and-moisture-migration-within-transformer-insulation-systems
- CIGRE TB 741, "Moisture measurement and assessment in transformer insulation – Evaluation of chemical methods and moisture capacitive sensors", Paris, August, 2018: https://e-cigre.org/publication/741-moisture-measurement-and-assessment-in-transformer-insulation-evaluation-of-chemical-methods-and-moisture-capacitive-sensors-2
- CIGRE TB 526, "Oxidation stability of insulating fluids", Paris, February, 2013: https://e-cigre.org/publication/526-oxidation-stability-of-insulating-fluids
- P.J. Burton, J. Graham, A.C. Hall, J.A. Laver, "Recent developments by CEGB to improve the prediction and monitoring of transformer performance", CIGRE Main Session, Paper 12-09, 1984: https://e-cigre.org/publication/12-09_1984-recent-developments-by-cegb-to-improve-the-prediction-and-monitoring-of-transformers-performance
- D.H. Shroff, A.W. Stannett, "A review of paper aging in power transformers", IEE Proceedings Vol. 132, Pt. C, No. 6, pp. 312-319, 1985.
- X. Chendong, "Monitoring paper insulation ageing by measuring furfural contents in oil", Proceedings of the 7th International Symposium on High Voltage Engineering, 1991.
- R.D. Stebbins, D.S. Myers, A.B. Shkolnik, "Furanic compounds in dielectric liquid samples: Review and update of diagnostic interpretation and estimation of insulation ageing", Proc. 7th Int. Conf. on Properties and Applications of Dielectric Materials, Nagoya, 2003.
- A. de Pablo, "New guidelines for furans analysis as well as dissolved gas analysis in oil-filled transformers Part 1: Furans analysis", CIGRE General Session, Paper 15/21/33-19, 1996: https://e-cigre.org/publication/152133-19_1996-new-guidelines-for-furans-analysis-as-well-as-dissolved-gas-analysis-in-oil-filled-transformers-parts-1--2
- L. Salgado, A. de Pablo, "About the interpretation methods of furans analyses", Euro TechCon, Bristol, 2016.
- J. Raith, Ch. Bonini, M. Scala, "Simulation of long-term transformer operation with a dynamic thermal, moisture and aging model", 5th International Colloquium Transformer Research and Asset Management, Opatija, 2019.
- V. Vasovic, J. Lukic, D. Mihajlovic, B. Pejovic, U. Radoman, Z. Radakovic, A. Orlovic, "Aging of transformer insulation - experimental transformers and laboratory models with different moisture contents: Part I – DP and furans aging profiles", IEEE Transactions on Dielectrics and Electrical Insulation, 26 (6), pp. 1840‑1846, ISSN: 1558-4135, DOI:10.1109/TDEI.2019.008183, 2019.
- V. Vasovic, J. Lukic, D. Mihajlovic, B. Pejovic, M. Milovanovic, U. Radoman, Z. Radakovic, "Aging of transformer insulation - experimental transformers and laboratory models with different moisture contents: Part II – Moisture distribution and aging kinetics", IEEE Transactions on Dielectrics and Electrical Insulation, 26 (6), pp. 1847-1852, ISSN: 1558-4135, DOI:10.1109/TDEI.2019.008184, 2019.
- CIGRE TB 673, "Guide on transformer transportation", Paris, 2016: https://e-cigre.org/publication/673-guide-on-transformer-transportation
- M. Hori, M. Nishioka, Y. Ikeda, K. Noguchi, K. Kajimura, H. Motoyama, T. Kawamura, "Internal winding failure due to resonance overvoltage in distribution transformer caused by winter lightning", IEEE Transactions on Power Delivery, vol. 21, no. 3, pp. 1600-1606, doi: 10.1109/TPWRD.2005.864046, July 2006.
- IEC 60076-14 (Annex B), "Liquid-immersed power transformers using high-temperature insulation materials", 2013.
- L. Pettersson, "Estimation of the remaining life of power transformers and their insulation", Electra, No. 133, 1990 (pp.65-71): https://e-cigre.org/publication/ELT_133-december-1990
- S. Miyazaki, M. Nakai, M. Yoshida, K. Aoki, "Rationalization and high precision of transformer lifetime evaluation method", accepted for publication in CIGRE e-session, 2020: https://e-cigre.org/publication/SESSION2020_A2-315
- IEC 60076-7, "Loading guide for oil-immersed power transformers", (2005) edition, 2005.
- CIGRE TB 738, "Ageing of liquid impregnated cellulose for power transformers", Paris, 2018: https://e-cigre.org/publication/738-ageing-of-liquid-impregnated-cellulose-for-power-transformers
- M. Duval, A. de Pablo, I. Atanasova-Hoehlein, M. Grisaru, "Significance and detection of very low degree of polymerization of paper in transformers", IEEE Electr. Insul. Magazine 33(1), pp. 31-38, 2017.
- CIGRE TB 227, "Life management techniques for power transformers", Paris, 2003: https://e-cigre.org/publication/227-life-management-techniques-for-power-transformers
- CIGRE TB 248, "Economics of transformer management", Paris, 2004: https://e-cigre.org/publication/248-economics-of-transformer-management
- CIGRE TB 494, "Furanic compounds for diagnosis", Paris, 2012: https://e-cigre.org/publication/494-furanic-compounds-for-diagnosis
- CIGRE TB 779, "Field experience with transformer solid insulation ageing markers", Paris, 2019: https://e-cigre.org/publication/779-field-experience-with-transformer-solid-insulation-ageing-markers
Further reading
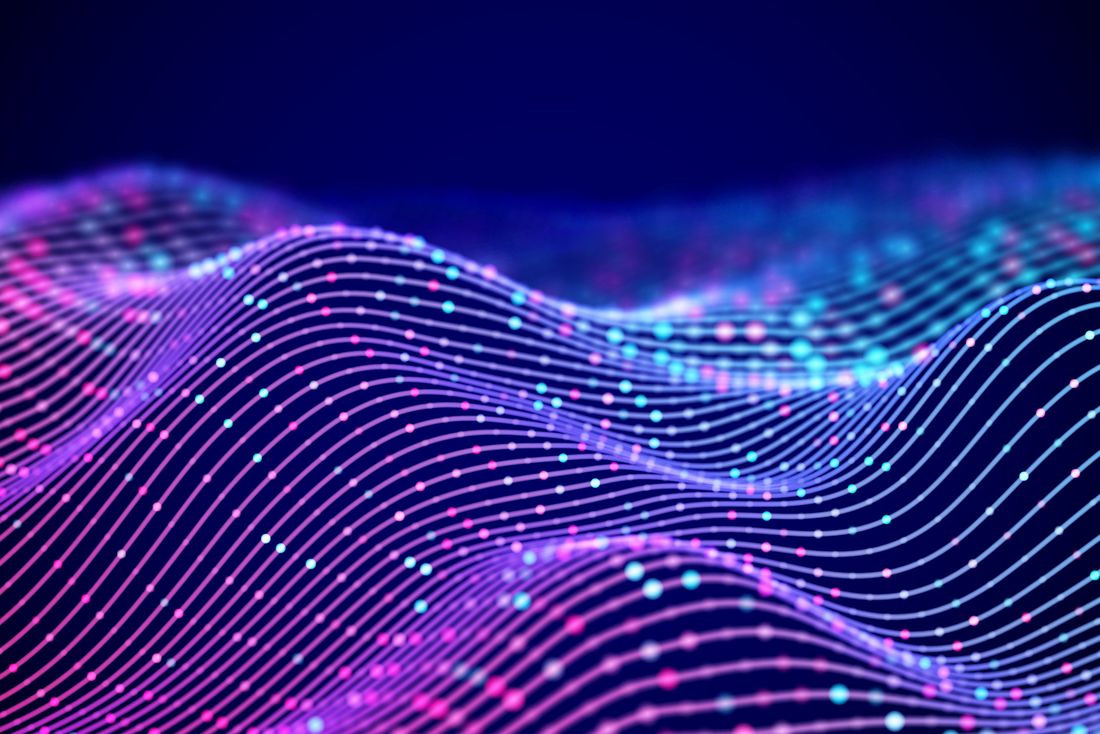
This article is a short summary of a longer and wider paper available for free. Readers are encouraged to reach out and read the full publication on e-cigre.
Thumbnail credit: Photo by Jan Huber on Unsplash
- [1] Aging effects due to drying in the transformer factory are currently investigated by CIGRE WG A2.64
- [2] The revised IEC 60076-7 (2018) discusses paper aging considering oxygen and water as well as the degradation model and parameters to use. Yet, the water content is assumed to be constant, which is not reality for moisture in cellulose during transformer operation, and in the appendix, it is unclear whether it is the hot-spot or the average water content.
- [3] Oil Quality includes Moisture (water content in oil), Acidity, DDF (Dielectric Dissipation Factor, also known as power factor), and IFT (Interfacial Tension).